Post by Life on Feb 21, 2021 5:17:55 GMT 5
THE ARCTIC PROXY
[1]
CAPTION: Preliminary results from a Smithsonian Institution project led by Scott Wing and Brian Huber, showing Earth's average surface temperature over the past 500 million years. For most of the time, global temperatures appear to have been too warm (red portions of line) for persistent polar ice caps. The most recent 50 million years are an exception. Image adapted from Smithsonian National Museum of Natural History.
More information in following link: www.science.org/content/article/500-million-year-survey-earths-climate-reveals-dire-warning-humanity
-----
MASS EXTINCTION EVENTS
[2]
-----
Emergence of dinosaurs
Dinosaurs emerged AFTER the Permian extinction event in the Carnian age of the Mesozoic time scale:
Theropoda outcompete Paracrocodylomorpha in the Triassic? - smoking gun in respiratory systems
-----
Mesozoic time scale for reference
[5]
---
Documented shifts in the Mesozoic
[6]
---
An alien world
---
Paleo-climatic conditions of the dinosaur-bearing Brazilian basins
NOTE: Contents of this section will be revisited/expanded from time-to-time.
Table II of [3] for reference:
[3]
[3]
[3]
[3]
Figure 7 in [6] for reference:
[6]
Figure 7 – (A) Early Cretaceous climatic zones as depicted in Scotese & McKerrow (1990); (B) Late Cretaceous climatic zones as modeled by Sellwood & Valdes (2006), drawn using GCM. In both maps, note the location of important dinosaur-bearing Brazilian basins: 1 – Bauru Basin, 2 = Parecis Basin, 3 – Recôncavo Basin, 4 – Araripe Basin, 5 = São Luis Basin, 6 – Amazonas Basin. Note that the paleoclimatic zone of the São Luis and Amazonas basins varied from arid to humid during the Cretaceous.
---
Paleo-climatic conditions of the Morrison Formation
NOTE: Contents of this section will be revisited/expanded from time-to-time.
Figures (1 - 2) in [8] for reference:
[8]
Figure 8 in [9] for reference:
[9]
Figure 8: Artist Reconstruction.
Dry season at the Mygatt-Moore Quarry showing Allosaurus feeding on a rotting, bug-infested carcass. Illustration by Brian Engh, dontmesswithdinosaurs.com (modified with permission from Drumheller et al., 2020).
-----
REFERENCES
[1] LINK: www.climate.gov/news-features/climate-qa/whats-hottest-earths-ever-been
[2] LINK: organismalbio.biosci.gatech.edu/biodiversity/mass-extinctions-and-climate-variability-2/
[3] Bittencourt, J. S., & Langer, M. C. (2011). Mesozoic dinosaurs from Brazil and their biogeographic implications. Anais da Academia Brasileira de Ciências, 83(1), 23-60.
[4] Hudgins, M. N., Uhen, M. D., & Hinnov, L. A. (2020). The evolution of respiratory systems in Theropoda and Paracrocodylomorpha, the end-Triassic extinction, and the role of Late Triassic atmospheric O2 and CO2. Palaeogeography, Palaeoclimatology, Palaeoecology, 545, 109638.
[5] Adopted from the following link: www.geosociety.org/GSA/Education_Careers/Geologic_Time_Scale/GSA/timescale/home.aspx
[6] Holz, M. (2015). Mesozoic paleogeography and paleoclimates–a discussion of the diverse greenhouse and hothouse conditions of an alien world. Journal of South American Earth Sciences, 61, 91-107.
[7] Parrish, J. T., Peterson, F., & Turner, C. E. (2004). Jurassic “savannah”—plant taphonomy and climate of the Morrison Formation (Upper Jurassic, Western USA). Sedimentary Geology, 167(3-4), 137-162.
[8] Engelmann, G. F., Chure, D. J., & Fiorillo, A. R. (2004). The implications of a dry climate for the paleoecology of the fauna of the Upper Jurassic Morrison Formation. Sedimentary Geology, 167(3-4), 297-308.
[9] McHugh, J. B., Drumheller, S. K., Riedel, A., & Kane, M. (2020). Decomposition of dinosaurian remains inferred by invertebrate traces on vertebrate bone reveal new insights into Late Jurassic ecology, decay, and climate in western Colorado. PeerJ, 8, e9510.
[10] Galli, K. G., Buchwaldt, R., Lucas, S. G., & Tanner, L. (2018). New chemical abrasion thermal ionization mass spectrometry dates from the Brushy Basin Member, Morrison Formation, western Colorado: implications for dinosaur evolution. The Journal of Geology, 126(5), 473-486.
[11] Drumheller, S. K., McHugh, J. B., Kane, M., Riedel, A., & D’Amore, D. C. (2020). High frequencies of theropod bite marks provide evidence for feeding, scavenging, and possible cannibalism in a stressed Late Jurassic ecosystem. Plos one, 15(5), e0233115.
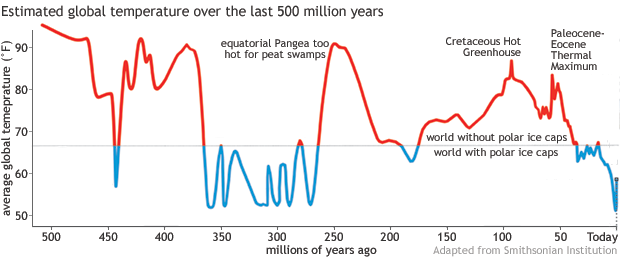
CAPTION: Preliminary results from a Smithsonian Institution project led by Scott Wing and Brian Huber, showing Earth's average surface temperature over the past 500 million years. For most of the time, global temperatures appear to have been too warm (red portions of line) for persistent polar ice caps. The most recent 50 million years are an exception. Image adapted from Smithsonian National Museum of Natural History.
A Smithsonian Institution project has tried to reconstruct temperatures for the Phanerozoic Eon, or roughly the last half a billion years. Preliminary results released in 2019 showed warm temperatures dominating most of that time, with global temperatures repeatedly rising above 80°F and even 90°F—much too warm for ice sheets or perennial sea ice. About 250 million years ago, around the equator of the supercontinent Pangea, it was even too hot for peat swamps!
Geologists and paleontologists have found that, in the last 100 million years, global temperatures have peaked twice. One spike was the Cretaceous Hot Greenhouse roughly 92 million years ago, about 25 million years before Earth’s last dinosaurs went extinct. Widespread volcanic activity may have boosted atmospheric carbon dioxide. Temperatures were so high that champsosaurs (crocodile-like reptiles) lived as far north as the Canadian Arctic, and warm-temperature forests thrived near the South Pole.
Another hothouse period was the Paleocene-Eocene Thermal Maximum (PETM) about 55-56 million years ago. Though not quite as hot as the Cretaceous hothouse, the PETM brought rapidly rising temperatures. During much of the Paleocene and early Eocene, the poles were free of ice caps, and palm trees and crocodiles lived above the Arctic Circle. ----[1]
Geologists and paleontologists have found that, in the last 100 million years, global temperatures have peaked twice. One spike was the Cretaceous Hot Greenhouse roughly 92 million years ago, about 25 million years before Earth’s last dinosaurs went extinct. Widespread volcanic activity may have boosted atmospheric carbon dioxide. Temperatures were so high that champsosaurs (crocodile-like reptiles) lived as far north as the Canadian Arctic, and warm-temperature forests thrived near the South Pole.
Another hothouse period was the Paleocene-Eocene Thermal Maximum (PETM) about 55-56 million years ago. Though not quite as hot as the Cretaceous hothouse, the PETM brought rapidly rising temperatures. During much of the Paleocene and early Eocene, the poles were free of ice caps, and palm trees and crocodiles lived above the Arctic Circle. ----[1]
More information in following link: www.science.org/content/article/500-million-year-survey-earths-climate-reveals-dire-warning-humanity
-----
MASS EXTINCTION EVENTS
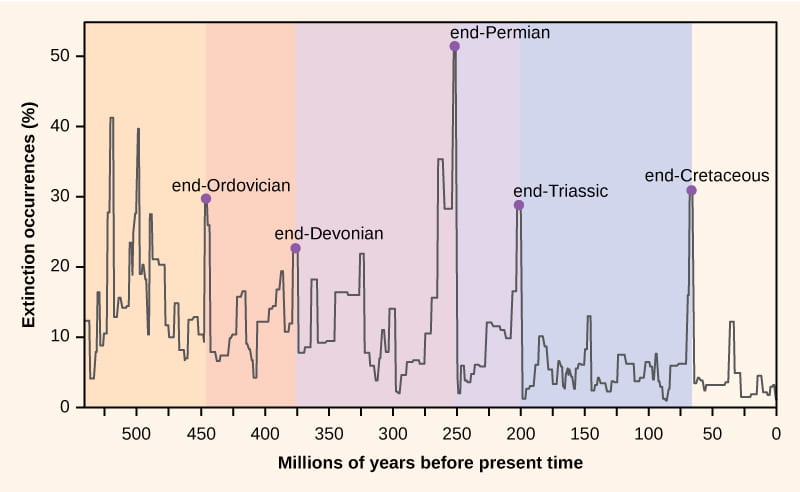
For some 3.7 billion years, life and the planet continued to evolve together, entwined in a mutual ebb and flow of evolutionary creation and cataclysmic devastation. Temperature records—compiled from miles-long ice cores filled with preserved gas bubbles and the remains of unicellular ocean-dwellers—can be compared to the fossil record to help scientists fill in the timeline of Earth’s history. The Cambrian explosion, for example, some 541 million years ago, saw a proliferation of complex life in the world’s oceans, possibly brought about by an increase in oxygen. The Permian extinction 252 million years ago, sparked by a rapid increase in volcanic activity and CO2 in the atmosphere, wiped out some 90 percent of all marine species and 70 percent of terrestrial species. ----[1]
-----
Emergence of dinosaurs
Dinosaurs emerged AFTER the Permian extinction event in the Carnian age of the Mesozoic time scale:
The Carnian witnessed the beginning of the dinosaur radiation, although taxa attributed to this clade are still rare (Langer et al. 2010, Nesbitt et al. 2009). This radiation has been studied in the light of the climatic changes registered in the end of the Triassic (Tucker and Benton 1982, Golonka and Ford 2000). In the Late Triassic strata of Rio Grande do Sul, these events are represented by an aridity increase during the Carnian (Zerfass et al. 2003), leading to the establishment of a mesoxerophytic flora dominated by the gymnosperm Dicroidium and concomitant faunal changing (Azevedo et al. 1990). ----[3]
Theropoda outcompete Paracrocodylomorpha in the Triassic? - smoking gun in respiratory systems
This study provides genera-level data on non-avian Theropoda and paracrocodylomorphs postcranial skeleton pneumaticity (PSP) using the Pneumaticity Index (PI) to quantify the evolution of pneumatization. The presence of foramina and fossae with continuous internal chambers as evidence of an avian-like respiratory system. Ancestral state reconstruction showed the evolutionary trends of pneumaticity in Theropoda and Paracrocodylomorpha, and a likely character state for the common ancestor between the two. O2 levels during the Triassic were low (11–14%) and correspond inversely with the high levels of atmospheric CO2. These findings also have implications for the predatory guild transition between Theropoda and Paracrocodylomorpha, as well as the decrease in diversity and disparity of paracrocodylomorphs through the Triassic. Increased PI values in Theropoda throughout the Late Triassic imply the presence of foramina in Theropoda were advantageous in the wavering O2 and CO2 environments however, fossae present in paracrocodylomorphs may not have been as advantageous as foramina, causing a reduction in pneumatization. The outcome resulting in a reduction in size of Paracrocodylomorpha with being replaced with more efficient Theropoda and becoming the subsidiary predator niche group. Presence of foramina in theropod skeletons before the changes in O2 and pCO2 levels suggest the character is an exaptation evolved for an earlier use for weight reduction, then later co-opted for the lower oxygen and CO2 environment. In summary, the rise of Theropoda may have been a multi-faceted event that persisted for millions of years, with the combination of the Carnian Pluvial Episode causing empty niches in Paracrocodylomorpha for Theropoda to occupy, the decreasing oxygen and wavering CO2 in the Triassic, and the selective advantage of foramina for efficient gas exchange in low oxygen levels may have played a critical role in the subsequent evolutionary success in Theropoda over Paracrocodylomorpha. ----[4]
-----
Mesozoic time scale for reference

---
Documented shifts in the Mesozoic

The Mesozoic Era was the time of the fragmenting of supercontinent Pangaea, implicating in dramatic changes concerning:
- paleocontinental positioning and shoreline configuration;
- intensity of volcanism and LIP emplacement;
- pattern of oceanic circulation;
- paleoclimate zonation;
- evolution of life.
The Era began under extreme severe “hothouse” conditions during Early Triassic, had mainly greenhouse conditions until Mid-Jurassic, when the first of a series of noticeable temperature drops occurred, inaugurating a phase of variable climates, where greenhouse conditions were followed by “cold snaps” or mini ice ages, but also eventual and short-lived hothouse conditions.
The mechanisms and interaction of the several climate-controlling factors such as the change in paleocontinental positioning, the opening of sea-ways modifying the oceanic circulation, the LIP emplacements enhancing CO2 and the impact events triggering “nuclear winters”, are still a controversy and debated matter. Some facts and events are closely related and might explain some paleoclimatic conditions, such as the global heating events and the atmospheric CO2 content. Other events, such as the Triassic humid pulses, are harder to explain.
The fact is that the major extinction events in Earth history can be found in the Mesozoic. The Phanerozoic extinction record suggests that a 10% generic turnover per stage can be considered as overall background extinctions or “normal faunal turnovers” (Keller, 2008). Hence, rates greater than 10% are considered extinction events, and such events did occur several times during the Mesozoic. The Triassic period records an important extinction event during the Norian/Rhaetian (> 30% extinction rate). Three noticeable extinctions occurred in the Jurassic (Pliensbachian, Callovian and Tithonian, all with extinction rates close to 20%), and three in the Cretaceous – during the Aptian (extinction rate ~15%), the Cenomanian (extinction rate ~18%) and specially the Maastrichian extinction that finished the reign of the dinosaurs on land and the ammonites in the oceans (extinction rate close to 45%).
Concerning Brazilian Mesozoic basins and tetrapod evolution, many questions arise if one compares the fossil record with the paleoenvironmental characterization of the different Mesozoic periods. For instance, the rock succession of the Recôncavo Basin probably records an important paleoclimatic change, from the cool greenhouse conditions in the Berriasian/Valanginian to warmer greenhouse regime of the Hauterivian/Barremian. Warm greenhouse to hothouse conditions had prevailed during the deposition of the basal part of the Bauru basin succession, while the upper part was deposited under cool greenhouse conditions. To what extend are these climatic variations linked to or, at least, the key control to the tetrapod evolution as recorded in those Brazilian basins? Brusatte et al. (2010) stated that it is essential to understand not only the climatic and environmental backdrop of dinosaur history, but also the absolute and relative ages of dinosaur fossils and whole dinosaur assemblages. A sound understanding of dinosaur evolution relies on geological data, which are fundamental to large-scale macroevolutionary studies. Especially for the Triassic and Early Jurassic, correlations between sedimentary successions on both local and global scales are extremely difficult, but should become a primary goal in future studies in dinosaur evolution. Fine-tuned studies, linking and correlating high resolution paleoclimatic data with full biostratigraphic range of dinosaur taxa in Brazilian basins, are still in their infancy, but are hopefully one of the outgrows of the 2013 symposium. ----[6]
- paleocontinental positioning and shoreline configuration;
- intensity of volcanism and LIP emplacement;
- pattern of oceanic circulation;
- paleoclimate zonation;
- evolution of life.
The Era began under extreme severe “hothouse” conditions during Early Triassic, had mainly greenhouse conditions until Mid-Jurassic, when the first of a series of noticeable temperature drops occurred, inaugurating a phase of variable climates, where greenhouse conditions were followed by “cold snaps” or mini ice ages, but also eventual and short-lived hothouse conditions.
The mechanisms and interaction of the several climate-controlling factors such as the change in paleocontinental positioning, the opening of sea-ways modifying the oceanic circulation, the LIP emplacements enhancing CO2 and the impact events triggering “nuclear winters”, are still a controversy and debated matter. Some facts and events are closely related and might explain some paleoclimatic conditions, such as the global heating events and the atmospheric CO2 content. Other events, such as the Triassic humid pulses, are harder to explain.
The fact is that the major extinction events in Earth history can be found in the Mesozoic. The Phanerozoic extinction record suggests that a 10% generic turnover per stage can be considered as overall background extinctions or “normal faunal turnovers” (Keller, 2008). Hence, rates greater than 10% are considered extinction events, and such events did occur several times during the Mesozoic. The Triassic period records an important extinction event during the Norian/Rhaetian (> 30% extinction rate). Three noticeable extinctions occurred in the Jurassic (Pliensbachian, Callovian and Tithonian, all with extinction rates close to 20%), and three in the Cretaceous – during the Aptian (extinction rate ~15%), the Cenomanian (extinction rate ~18%) and specially the Maastrichian extinction that finished the reign of the dinosaurs on land and the ammonites in the oceans (extinction rate close to 45%).
Concerning Brazilian Mesozoic basins and tetrapod evolution, many questions arise if one compares the fossil record with the paleoenvironmental characterization of the different Mesozoic periods. For instance, the rock succession of the Recôncavo Basin probably records an important paleoclimatic change, from the cool greenhouse conditions in the Berriasian/Valanginian to warmer greenhouse regime of the Hauterivian/Barremian. Warm greenhouse to hothouse conditions had prevailed during the deposition of the basal part of the Bauru basin succession, while the upper part was deposited under cool greenhouse conditions. To what extend are these climatic variations linked to or, at least, the key control to the tetrapod evolution as recorded in those Brazilian basins? Brusatte et al. (2010) stated that it is essential to understand not only the climatic and environmental backdrop of dinosaur history, but also the absolute and relative ages of dinosaur fossils and whole dinosaur assemblages. A sound understanding of dinosaur evolution relies on geological data, which are fundamental to large-scale macroevolutionary studies. Especially for the Triassic and Early Jurassic, correlations between sedimentary successions on both local and global scales are extremely difficult, but should become a primary goal in future studies in dinosaur evolution. Fine-tuned studies, linking and correlating high resolution paleoclimatic data with full biostratigraphic range of dinosaur taxa in Brazilian basins, are still in their infancy, but are hopefully one of the outgrows of the 2013 symposium. ----[6]
---
An alien world
The rifting of Pangaea occurred during the Mesozoic, eventually fragmenting this supercontinent into several plates which will form the modern continents. This break-up had profound consequences not only for the paleocontinental configuration, but also for paleoclimates and for the evolution of life; because it significantly modified the paleoposition and shoreline configurations of the land masses, generated huge epicontinental seas and altered profoundly the oceanic circulation.
Due to its unique paleoclimatic conditions, with several times of very high temperatures (mean global temperature > 25oC) alternating with less warmer intervals and even with short-lived ice-ages, the Mesozoic Earth was, in comparison to the present, an “alien world”, paraphrasing Sellwood & Valdes (2006). Fischer (1982) introduced the nowadays widely used terms ‘greenhouse’ and ‘icehouse’ to characterize the two major climatic states of the Earth during the Phanerozoic. Kidder & Worsley (2010, 2012) proposed that there are actually three basic states for Earth climate: Icehouse, Greenhouse (subdivided into Cool and Warm states), and Hothouse. The ‘Icehouse’ (IH) displays polar ice, strong latitudinal temperature gradient (50–60°C) and alternating glacial–interglacial episodes in response to orbital forcing. This climatic state apparently did not occur during the whole Mesozoic. The ‘Cool Greenhouse” (GH-c) shows some polar ice and alpine glaciers, but no ice streams calving icebergs into the ocean. Global average temperatures are thought to range between 21° and 24°C. Atmospheric CO2 levels are thought to have been between 2 and 4 times those of today (600–1,200 ppmv). The high to low latitude temperature gradient is weaker that in the icehouse (ca. 40°C). The ‘Warm Greenhouse’ (GH-w) is devoid of any polar ice. Global average temperatures might have ranged from 24° to 30°C. Kidder and Worsley (2012) believe that atmospheric CO2 levels ranged between 4 and 16 times the present ones (1,200–4,800 ppmv) during GH-w stages. Seasonal reversals of the atmospheric pressure systems around the poles should have occured. The lower latitudinal temperature gradients (< 34°C) thus mean reduced winds, and the warmer ocean would absorb even less oxygen. Isolated basins could become bottom oxygen-depleted. The “Hothouse” (HH) condition is relatively short-lived (< 1 to ca. 3 Ma) and results from anomalously large inputs of CO2 into the atmosphere during the formation of Large Igneous Provinces (LIPs), when atmospheric CO2 concentrations may rise above 16 times (4,800 ppmv).
The Mesozoic Earth had been experiencing the cool greenhouse, warm greenhouse and the hothouse climatic states. Hence, Mesozoic climate was very variable, what is corroborated by several global-scale studies. For instance, Veizer et al. (2000) demonstrated the variability of the Mesozoic global climate relying on a study on atmospheric CO2 and global tropical sea surface temperatures anomalies (TSSTA), reporting positive anomalies for the Early to Middle Triassic and for the Late Cretaceous, and negative anomalies during Early Jurassic and specially for the Mid-Jurassic, when the mean surface temperatures were 4oC higher than today.
Global sea levels throughout the Mesozoic were generally much higher than at present, with exceptions for the sea level lowstand in the Early Induan (i.e, the beginning of the Triassic, cf. Haq et al. 1988) and two noticeable lowstands in the early Jurassic (Hettangian/Sinemurian) and in the early Cretaceous (Valanginian) (Haq, 2014).
Mesozoic atmospheric oxygen content was also different when compared to current values. Theoretical calculations, based on both chemical and isotopic composition of sedimentary rocks and admitting a wide margin of error (about +10%), indicate that atmospheric O2 had been varying between 15 and 25% (Campbell & Allen, 2008, Royer, 2014). The transition from Permian to Triassic is marked by a huge drop of the atmospheric oxygen content, falling from ~30% to less than 15% according to some authors (e.g. Dudley, 2000; Berner et al., 2003). During Induan/Olenekian (Early Triassic), the O2 content was about 15%, then it increasing to 20% (i.e. close to the present-day level) until the Early Jurassic and finally to 25% toward the Late Cretaceous (Berner & Canfield, 1989; Berner et al., 2003; Holland, 2006).
Why did the Mesozoic have such a different and overall hotter climate when compared, for instance, to the present day world? The main factors are:
(1) the paleocontinental configuration: the assembling of a supercontinent, forming an almost pole-to-pole continuous land mass, is one of the climate-driving factors. The combination of a gigantic land mass (Pangaea covered an approximate surface of 130 million km2) and a lower global sea level (associated with reduced rates of tectonic movement) resulted in an arid climate during the Early Mesozoic, as stated by Frakes (1979). Parrish (1993) assigned to the Triassic the maximum development of megamonsoons, due to an almost symmetrical position of Pangaea over the equator. This megamonsoonal regime would have caused the displacement of the arid belt towards the poles, causing a decrease of the precipitation/evaporation rate in the mid-latitudes of Pangaea interiors, where huge semi-arid to arid belts characterized that period. The rifting of the supercontinent began during the Triassic, and the major break-up of Pangaea took place during the Jurassic (when Laurasia rifted from Gondwana and Africa separated from Antarctica/Australia); and continued during the Cretaceous (when Africa and South America rifted apart, and Greenland separated from North America). The break-up and the consequent continental drift modified the paleoposition and shoreline configurations of the land masses, generated huge epicontinental seas, separated land masses by interior sea ways, and altered profoundly the oceanic circulation. These factors helped controlling climate change during Middle and Late Mesozoic.
(2) the degassing process linked to plate tectonics: intense sea-floor spreading enhanced greenhouse conditions thanks to degassing of H2S, CH4 and CO2 (Seton et al., 2009; Royer, 2014), and rifting events are frequently linked to flood basalts and to the emplacements of large igneous provinces (LIPs) both continental and oceanic. It is a climate-controlling factor: since during these events the short term cooling is followed by warming induced by CO2 degassing and enhanced greenhouse effect on climate (e.g. Kidder & Worsley, 2010; Chui & Komp, 2014). During the entire Mesozoic the CO2 levels were relatively high (1,000–2,000 ppmv), with transient excursions to even higher values (>2,000 ppmv; Retallack, 2001), and extremely elevated CO2 concentrations at the Triassic/Jurassic boundary (Steinthorsdottir et al., 2011). Times of high atmospheric CO2 and the consequent warm-wet paleoclimatic conditions led to the so-called “greenhouse crises” (Retallack, 2013), which are belived to have played a role in mass extinctions and long-term evolutionary trends during the entire Phanerozoic (Retallack, 2009). Greenhouse gases would have not only disrupted life on land with warmer and wetter climates, but with spreading tropical pathogens, long-distance plant and animal migrations, sea level rise, low-oxygenated ground water and water acidification. Atmospheric oxygen depleted by massive emissions of CH4 and H2S (Berner, 2006) would have challenged animals with pulmonary edema, high elevations might have become uninhabitable, later reducing habitat for many animals (Huey & Ward, 2005), and animals with small–medium body size and short muscular limbs (e.g. Lystrosaurus), would be the evolutionary outcome of such a greenhouse crisis (Retallack et al., 2003).
The evolution of terrestrial vertebrates seemingly was another climate-controlling factor. During Mesozoic, herbivorous vertebrates display an evolutionary trend towards gigantism; hence, methane emissions from that kind of reptilian biotas are also considered a climate-controlling factor: the vast herds of grazing reptiles might have released enhanced methane content in the atmosphere and therefore elevated the warm to hot greenhouse conditions of the Mesozoic world (Wilkinson et al., 2012).
(3) the impact events: the collision of huge asteroids produces a huge amount of ejecta which is smeared through atmospheric circulation over huge areas or even globally, causing the so-called “impact winter“ which is a period of prolonged cold weather resulting from blocked sunlight (e.g. Covey et al., 1994; Chapman & Morrison, 1994), hence, inducing severe ecological alterations that could lead to extinction (e.g. Raup, 1992). ----[6]
Due to its unique paleoclimatic conditions, with several times of very high temperatures (mean global temperature > 25oC) alternating with less warmer intervals and even with short-lived ice-ages, the Mesozoic Earth was, in comparison to the present, an “alien world”, paraphrasing Sellwood & Valdes (2006). Fischer (1982) introduced the nowadays widely used terms ‘greenhouse’ and ‘icehouse’ to characterize the two major climatic states of the Earth during the Phanerozoic. Kidder & Worsley (2010, 2012) proposed that there are actually three basic states for Earth climate: Icehouse, Greenhouse (subdivided into Cool and Warm states), and Hothouse. The ‘Icehouse’ (IH) displays polar ice, strong latitudinal temperature gradient (50–60°C) and alternating glacial–interglacial episodes in response to orbital forcing. This climatic state apparently did not occur during the whole Mesozoic. The ‘Cool Greenhouse” (GH-c) shows some polar ice and alpine glaciers, but no ice streams calving icebergs into the ocean. Global average temperatures are thought to range between 21° and 24°C. Atmospheric CO2 levels are thought to have been between 2 and 4 times those of today (600–1,200 ppmv). The high to low latitude temperature gradient is weaker that in the icehouse (ca. 40°C). The ‘Warm Greenhouse’ (GH-w) is devoid of any polar ice. Global average temperatures might have ranged from 24° to 30°C. Kidder and Worsley (2012) believe that atmospheric CO2 levels ranged between 4 and 16 times the present ones (1,200–4,800 ppmv) during GH-w stages. Seasonal reversals of the atmospheric pressure systems around the poles should have occured. The lower latitudinal temperature gradients (< 34°C) thus mean reduced winds, and the warmer ocean would absorb even less oxygen. Isolated basins could become bottom oxygen-depleted. The “Hothouse” (HH) condition is relatively short-lived (< 1 to ca. 3 Ma) and results from anomalously large inputs of CO2 into the atmosphere during the formation of Large Igneous Provinces (LIPs), when atmospheric CO2 concentrations may rise above 16 times (4,800 ppmv).
The Mesozoic Earth had been experiencing the cool greenhouse, warm greenhouse and the hothouse climatic states. Hence, Mesozoic climate was very variable, what is corroborated by several global-scale studies. For instance, Veizer et al. (2000) demonstrated the variability of the Mesozoic global climate relying on a study on atmospheric CO2 and global tropical sea surface temperatures anomalies (TSSTA), reporting positive anomalies for the Early to Middle Triassic and for the Late Cretaceous, and negative anomalies during Early Jurassic and specially for the Mid-Jurassic, when the mean surface temperatures were 4oC higher than today.
Global sea levels throughout the Mesozoic were generally much higher than at present, with exceptions for the sea level lowstand in the Early Induan (i.e, the beginning of the Triassic, cf. Haq et al. 1988) and two noticeable lowstands in the early Jurassic (Hettangian/Sinemurian) and in the early Cretaceous (Valanginian) (Haq, 2014).
Mesozoic atmospheric oxygen content was also different when compared to current values. Theoretical calculations, based on both chemical and isotopic composition of sedimentary rocks and admitting a wide margin of error (about +10%), indicate that atmospheric O2 had been varying between 15 and 25% (Campbell & Allen, 2008, Royer, 2014). The transition from Permian to Triassic is marked by a huge drop of the atmospheric oxygen content, falling from ~30% to less than 15% according to some authors (e.g. Dudley, 2000; Berner et al., 2003). During Induan/Olenekian (Early Triassic), the O2 content was about 15%, then it increasing to 20% (i.e. close to the present-day level) until the Early Jurassic and finally to 25% toward the Late Cretaceous (Berner & Canfield, 1989; Berner et al., 2003; Holland, 2006).
Why did the Mesozoic have such a different and overall hotter climate when compared, for instance, to the present day world? The main factors are:
(1) the paleocontinental configuration: the assembling of a supercontinent, forming an almost pole-to-pole continuous land mass, is one of the climate-driving factors. The combination of a gigantic land mass (Pangaea covered an approximate surface of 130 million km2) and a lower global sea level (associated with reduced rates of tectonic movement) resulted in an arid climate during the Early Mesozoic, as stated by Frakes (1979). Parrish (1993) assigned to the Triassic the maximum development of megamonsoons, due to an almost symmetrical position of Pangaea over the equator. This megamonsoonal regime would have caused the displacement of the arid belt towards the poles, causing a decrease of the precipitation/evaporation rate in the mid-latitudes of Pangaea interiors, where huge semi-arid to arid belts characterized that period. The rifting of the supercontinent began during the Triassic, and the major break-up of Pangaea took place during the Jurassic (when Laurasia rifted from Gondwana and Africa separated from Antarctica/Australia); and continued during the Cretaceous (when Africa and South America rifted apart, and Greenland separated from North America). The break-up and the consequent continental drift modified the paleoposition and shoreline configurations of the land masses, generated huge epicontinental seas, separated land masses by interior sea ways, and altered profoundly the oceanic circulation. These factors helped controlling climate change during Middle and Late Mesozoic.
(2) the degassing process linked to plate tectonics: intense sea-floor spreading enhanced greenhouse conditions thanks to degassing of H2S, CH4 and CO2 (Seton et al., 2009; Royer, 2014), and rifting events are frequently linked to flood basalts and to the emplacements of large igneous provinces (LIPs) both continental and oceanic. It is a climate-controlling factor: since during these events the short term cooling is followed by warming induced by CO2 degassing and enhanced greenhouse effect on climate (e.g. Kidder & Worsley, 2010; Chui & Komp, 2014). During the entire Mesozoic the CO2 levels were relatively high (1,000–2,000 ppmv), with transient excursions to even higher values (>2,000 ppmv; Retallack, 2001), and extremely elevated CO2 concentrations at the Triassic/Jurassic boundary (Steinthorsdottir et al., 2011). Times of high atmospheric CO2 and the consequent warm-wet paleoclimatic conditions led to the so-called “greenhouse crises” (Retallack, 2013), which are belived to have played a role in mass extinctions and long-term evolutionary trends during the entire Phanerozoic (Retallack, 2009). Greenhouse gases would have not only disrupted life on land with warmer and wetter climates, but with spreading tropical pathogens, long-distance plant and animal migrations, sea level rise, low-oxygenated ground water and water acidification. Atmospheric oxygen depleted by massive emissions of CH4 and H2S (Berner, 2006) would have challenged animals with pulmonary edema, high elevations might have become uninhabitable, later reducing habitat for many animals (Huey & Ward, 2005), and animals with small–medium body size and short muscular limbs (e.g. Lystrosaurus), would be the evolutionary outcome of such a greenhouse crisis (Retallack et al., 2003).
The evolution of terrestrial vertebrates seemingly was another climate-controlling factor. During Mesozoic, herbivorous vertebrates display an evolutionary trend towards gigantism; hence, methane emissions from that kind of reptilian biotas are also considered a climate-controlling factor: the vast herds of grazing reptiles might have released enhanced methane content in the atmosphere and therefore elevated the warm to hot greenhouse conditions of the Mesozoic world (Wilkinson et al., 2012).
(3) the impact events: the collision of huge asteroids produces a huge amount of ejecta which is smeared through atmospheric circulation over huge areas or even globally, causing the so-called “impact winter“ which is a period of prolonged cold weather resulting from blocked sunlight (e.g. Covey et al., 1994; Chapman & Morrison, 1994), hence, inducing severe ecological alterations that could lead to extinction (e.g. Raup, 1992). ----[6]
---
Paleo-climatic conditions of the dinosaur-bearing Brazilian basins
NOTE: Contents of this section will be revisited/expanded from time-to-time.
Mesozoic periods | Basins | Description | Formations and dinosaurian footprints and/or impressions | Classification |
Triassic | The Paraná Basin | Triassic dinosaurs in Brazil were exclusively collected from rocks of the Santa Maria and the overlying Caturrita formations, in central Rio Grande do Sul (Fig. 1, Table I, II, Langer et al. 2007a). These units belong to the Rosário do Sul Group (Andreis et al. 1980), which corresponds to the Gondwana Supersequence II of the Paraná Basin (Milani 2004). Recent studies explain the origin of this Supersequence as the infilling of tectonically restricted half-graben depocenters (Milani 2001, 2004, Zerfass et al. 2003). The Santa Maria Formation is composed predominantly of red mudstones (Andreis et al. 1980, Scherer et al. 2000, Silva et al. 2003) and encompasses two distinct faunal associations of Ladinian and Carnian age (Barberena 1977, Langer et al. 2007a), as also suggested by stratigraphic studies (Zerfass et al. 2003). The Ladinian sequence represents a more humid phase within a generally seasonal and semi-arid climate. Fossil tetrapods were preserved either in lacustrine environments or in flood plains of an anastomosed fluvial system (Scherer et al. 2000, Zerfass et al. 2003). The Carnian sequence was formed in a similar environment, but the presence of aeolian deposits suggests an increased aridity (Zerfass et al. 2003). The coarser-grained, red sandstones of the Caturrita Formation represent the establishment of a perennial braided fluvial system, hinting at the return of more humid conditions (Holz and Scherer 2000, Zerfass et al. 2003). The age of this unit has been generally accepted as Norian (Rubert and Schultz 2004, Bonaparte et al. 2007, Langer et al. 2007a) [3]. The only possible dinosaur recovered from the Ladinian sequence of the Santa Maria Formation is Spondylosoma absconditum Huene, 1942 (Table II) [3]. The Carnian witnessed the beginning of the dinosaur radiation, although taxa attributed to this clade are still rare (Langer et al. 2010, Nesbitt et al. 2009). This radiation has been studied in the light of the climatic changes registered in the end of the Triassic (Tucker and Benton 1982, Golonka and Ford 2000). In the Late Triassic strata of Rio Grande do Sul, these events are represented by an aridity increase during the Carnian (Zerfass et al. 2003), leading to the establishment of a mesoxerophytic flora dominated by the gymnosperm Dicroidium and concomitant faunal changing (Azevedo et al. 1990) [3]. | Sauropodomorpha [3] Silesauridae [3] Theropoda [3] Herrerasauridae [3] | |
Jurassic | ||||
Cretaceous | The Recôncavo Basin | The Recôncavo Basin, located in northeastern Brazil, is a typical example of a continental rift basin. It is the southern part of the Recôncavo–Tucano–Jatobá rift system, which developed during the break-up of Gondwana, during the Late Jurassic and Early Cretaceous. The basin is characterized by asymmetric graben structures comprising three fault-delimited compartments (southern, central and northern), and is exposed over an area of 11,500km2. In the depocenter, a maximum basinal infilling of up to 6,500 meters is reached. The rift sequence is characterized by fluvial–deltaic, lacustrine and alluvial fan deposits (Holz et al., 2014), and according to the paleoclimatic zonation shown in Figure 7, the basin was located in an arid zone during the entire Cretaceous. However, correlation with the global temperature curve (Appendix 1) suggests that the Recôncavo Basin developed during the aforementioned noticeable global temperature increase mapped for the Valanginian-Hauterivian transition (Appendix 1), indicating that the main rift sediments were deposited under warm greenhouse conditions [6]. | The dinosaur fossil record is poor up to date, only isolated vertebrae are recorded (Bitencourt & Langer, 2011), without any taphonomic information [6]. | |
Cretaceous | The São Luis/Grajaú Basin | The São Luis/Grajaú Basin is located in the equatorial portion of Brazil and covers an area of 250,000km2. Its genesis is related to the processes of continental rupture and consequent formation of the South Atlantic rift initiated during the Late Jurassic and Early Cretaceous (Góes and Rossetti, 2001). The sedimentary record consists almost entirely of Cretaceous rocks that can be divided into three depositional sequences sensu Rossetti (2001). Sequence S1 accumulated during the Late Aptian and Early Albian, and includes sandstones, shales and limestones deposited in shallow marine, lacustrine and fluvio-deltaic environments that make up the Grajaú and Codó Formations. Sequence S2, of Early–Middle Albian age, consists of sandstone and mudstones of shallow marine and fluvio-deltaic environments that form the Itapecuru and Codó formations. Sequence S3 developed between the Middle Albian and Cenomanian. It comprises sandstones and mudstones referred to the Alcântara and Cujupe Formations. This is the most important dinosaur-bearing unit of that basin, with a taphocoenosis represented by thousands of logs, bone fragments and teeth. Thin section analysis of the rock matrix revealed fine-grained fragments and even “bone flour”, evidencing intense reworking of the bones prior to final burial. The dinosaur taphocoenosis of the Alcântara Formation are actually high-order sequence boundaries coinciding with erosive trangressive surfaces. They were produced by sea-level fall, when the dinosaur bones were transported basinwards in a highly selective manner, and were reworked during the subsequent transgressive events, resulting in highly chaotic and mixed-up fossil deposits. It is a rare example of dinosaur fossils marking transgressive surfaces within an incised valley infill (Holz, 2003). [6]. | Most of the assemblage represents a continental paleocommunity similar to the coeval northern African fauna, including dinosaurs such as Carcarodontosaurus, Spinosaurus, titanosaurs and a rebbachisaurid form (Medeiros et al., 2007). The dinosaurian fauna lived in an arid to semi-arid environment, according to paleoenvirommental discussion by Medeiros et al. (ibidem) and Lindoso et al. (2013); that is concurrent with the global paleoclimatic characterization of the Early Cretaceous as shown in figure 7A [6]. | Titanosuaria [3] Diplodocoidea [3] Carcharodontosauridae [3] Spinosauridae [3] Deinonychosauria [3] |
Cretaceous | The Araripe Basin | The Araripe Basin, also located in the northeastern region of Brazil, is a small interior rift basin (area of ~9000km2) where Assine (2007) identified four unconformity-bounded units: a Palaeozoic Sequence (represented by the alluvial Mauriti Formation), an Early Jurassic Pre-Rift Supersequence (Brejo Santo and Missão Velha Formations), a Barremian to Hauterivian Rift Supersequence (formed by the Abaiara Formation); and a Albian/Aptian Post-Rift Supersequence, formed by the Barbalha, Santana, Araripina and Exu Formations [6]. | Dinosaur-bearing strata are linked to the Albian/Aptian Santana Formation of the Post-Rift supersequence, under warm greenhouse conditions, with global temperature peaking during the Albian/Cenomanian (cf. Zhou et al., 2012). All dinosaurs from the Santana Formation are theropods and at least four different clades were identified (Bitencourt & Langer, 2011). Fragments of rostrum, skulls, ribs, isolated teeth and feathers are preserved in carbonate concretions from both fluvial and lacustrine strata [6]. | Enantiornithes [3] Compsognathidae [3] Tyrannoraptora [3] Spinosauridae [3] |
Cretaceous | The Parecis Basin | The Parecis Basin is a large intracratonic sag (~500,000km2), with an E-W elongation, located in central Brazil, with a sedimentary succession up to 6km thick. The oldest sedimentary rocks are Neoproterozoic to Ordovician and Siluro–Devonian, while the youngest ones are Mesozoic and Tertiary, but very little chronostratigraphic information is available for this basin (Goldberg et al., 2013). Actually, it is one of the least explored sedimentary basins of Brazil. According to its paleocontinental location, the area of the basin had displayed arid climate throughout the entire Cretaceous (Fig.7) [6]. | Cretaceous deposits of the northwestern margin of the Paraná Basin crop out in the state of Mato Grosso (Fig. 1). Their strata have been referred to the Cambambe Formation (sensu Weska et al. 1996), which was deposited in a fluviolacustrine system associated to distal parts of alluvial fans under a semi-arid to extremely arid climate (Rosa et al. 1991, Weska 2006). This unit has been traditionally referred to the Bauru Group (FrancoRosas et al. 2004, Lacerda Filho et al. 2004), but recent work suggests that all Cretaceous rocks deposited south of the Paraguai fold belt (Almeida 1984) in Mato Grosso should be included in the Parecis Group (Weska 2006). These deposits accumulated on a crust depression formed by tectonic distension events related to the magmatic plume of Trindade (Weska 2006). The basalts of the Paredão Grande Formation, at the base of the Parecis Group, were dated 83.9 ± 0.4 Ma (Gibson et al. 1997), i.e. Late Santonian (Gradstein and Ogg 2004). This geophysical and other paleontological evidence (FrancoRosas et al. 2004) suggest a Campanian-Maastrichtian age for the dinosaur fauna of the Parecis Group [3]. Pieces of dinosaur post-cranium and isolated teeth, vertebrae and tibia are recorded for the Late Cretaceous Parecis Group (Bittencourt & Langer, 2011), but no piece of taphonomic information is available so far [6]. | Titanosauria [3] Abelisauridae [3] Deinonychosauria [3] |
Cretaceous | The Bauru Basin | The Bauru Basin is an intracratonic basin with a Late Cretaceous (Cenomanian to Maastrichtian) infilling [6]. During that period, a broad zone with excessive evaporation extends from the equator to beyond 30°S, embracing central Africa and the central parts of South America, where the Bauru Basin is located. The predominantly arid climate of that zone is corroborated by several faciological and taphonomical evidence (e.g. Carvalho et al., 2010, Araujo & Marinho, 2013) and fits with the global Late Cretaceous climate characterization (Fig.7) [6]. | The Cretaceous of the Bauru Group (sensu Soares et al. 1980) has so far provided the most significant record of Brazilian dinosaurs (Kellner and Campos 2000). Its strata are also referred to as Bauru Supersequence (Fig. 1, sensu Milani 2001) and were formed in an extensive subsidence area created by the lithostatic load of the Early Cretaceous basalts of the Serra Geral Formation, upon which the Bauru beds are discordantly deposited (Fulfaro and Perinotto 1996, Fernandes and Coimbra 2000, Milani 2004) [3]. The Bauru dinosaurs (Table I, II) are commonly found in localities of western São Paulo and Minas Gerais, where the Adamantina and Marília formations are widely known for their rich fossiliferous content (Bertini et al. 2003, Candeiro et al. 2006a, 2008) [3]. Dinosaur remains, mostly from Adamantina and Marília Formations (Bauru Group), are allocthonous, preserved in deposits of alluvial fans and braided river systems, where periodical flash-flood events reworked previously exposed soil sediments (caliche) [6]. | Titanosuaria [3] Carcharodontosauridae [3] Abelisauridae [3] Deinonychosauria [3] Enantiornithes [3] |
Table II of [3] for reference:




Figure 7 in [6] for reference:

Figure 7 – (A) Early Cretaceous climatic zones as depicted in Scotese & McKerrow (1990); (B) Late Cretaceous climatic zones as modeled by Sellwood & Valdes (2006), drawn using GCM. In both maps, note the location of important dinosaur-bearing Brazilian basins: 1 – Bauru Basin, 2 = Parecis Basin, 3 – Recôncavo Basin, 4 – Araripe Basin, 5 = São Luis Basin, 6 – Amazonas Basin. Note that the paleoclimatic zone of the São Luis and Amazonas basins varied from arid to humid during the Cretaceous.
---
Paleo-climatic conditions of the Morrison Formation
NOTE: Contents of this section will be revisited/expanded from time-to-time.
Mesozoic periods | Description | Dinosaurian footprint and/or impressions | Classification |
Jurassic | The Morrison Formation contains six plant taphofacies: Wood, whole-leaf, leaf-mat, root, common carbonaceous debris, and rare carbonaceous debris. None of these taphofacies is common in the Morrison; particularly striking is the paucity of wood, even in more reducing environments. The flora of the Morrison Formation is distinct in the Kimmeridgian and Tithonian parts of the section. The plant taphofacies are consistent with a predominantly herbaceous vegetation. Evidence from the plant taphofacies, floras, and sedimentology of the Morrison is consistent with a warm, seasonal, semi-arid climate throughout, changing from dry semi-arid to humid semi-arid from the Kimmeridgian to the Tithonian parts of the formation [7]. The flora of the Morrison Formation was predominantly herbaceous and, in the Kimmeridgian part of the formation, resembled that of modern savannahs in which trees are not abundant and are most common in the riparian environments. Although the vegetation in the Tithonian part of the Morrison was still predominantly herbaceous, it included elements that are consistent with a slightly more humid climate. We propose that the climate was slightly wetter in the Tithonian because either precipitation was higher or cooling led to lower evaporation rates, or both [7]. The taxonomic composition of the floras in the Kimmeridgian and Tithonian parts of the Morrison Formation is strikingly different, and it is therefore misleading to consider the flora of the entire formation as a single vegetational unit [7]. | Efficient Sauropods vs. others and niche partitions In light of diverse geological evidence that indicates a seasonal, semiarid climate for the time of deposition of the Morrison Formation, one can assume these general environmental conditions for the purpose of reconstructing the ancient ecosystem. Wet environments that preserved plant fossils and some invertebrates and small vertebrates in the Morrison can be interpreted as representing local conditions limited in space and/or time. These elements of the biota and the smaller dinosaurs were probably restricted to such wetland areas at times of environmental stress [8]. A diverse fauna of large, herbivorous, sauropod dinosaurs ranged throughout the environment. Although this seems to be inconsistent with an environment with sparse resources, large size confers physiologic advantages that are adaptive for just such conditions. The scaling effect of large size makes large herbivores very efficient relative to their size in needing proportionately less food and food of poorer quality than smaller herbivores. They can also survive starvation longer and travel more efficiently to reach widely separated resource patches. Although few in number at any time, the sauropod dinosaurs are locally abundant and seemingly ubiquitous in the fossil record of the Morrison Formation because of overrepresentation of their highly preservable remains in an attritional fossil assemblage [8]. Sauropod dinosaur species partitioned the food resources by varied feeding strategies, possibly including vertical stratification of browsing levels. High-browsing brachiosaurs were probably relatively rare, while camarasaurs browsing on course vegetation at intermediate height were more common. Diplodocids browsed on the low-growing herbaceous plants. Predatory dinosaurs of varying size occupied the same range as their prey species [8]. The very large predatory dinosaurs may have specialized as scavengers on the large carcasses of sauropods. This habitat might expand during a wet season or be reduced to small, widely separated refugia during periods of severe drought. Relatively small populations of adult sauropods utilized very large geographic ranges, traveling long distances between resource areas, especially during times of drought. Other, smaller, herbivorous dinosaurs, including juvenile sauropods, may have been more specialized feeders and ranged less widely than the adult sauropods [8]. In his study of the paleoecology of the Morrison, Foster (1998) analyzed the various ecological guilds represented in the Morrison with respect to taxonomic diversity and adult body mass. He found that for herbivores, the greatest diversity occurs at very large and very small body size, with much lower diversity in intermediate sizes. He offered two possible explanations for this pattern: that the intermediate body size classes may have been filled by the juveniles of the very large species (sauropods), or that the intermediate size herbivores may have been more vulnerable to predation by moderate to large predators [8]. This size distribution of species might also be explained as a result of two successful strategies for survival in an environment such as that postulated for the Morrison. At times of limited availability of water, the large sauropods could survive by traveling from one resource patch to another. Smaller species were confined to those small areas with persistent water resources, but smaller body size enabled them to maintain larger populations and greater diversity, and rapidly expand their populations when resources were more abundant. Times of severe drought would be especially harsh on herbivores of intermediate body size. Sauropods would have to grow through such an intermediate size, and would be especially vulnerable to severe drought at that point in their development [8]. --- The Morrison mammals are not instructive The mammals of the Morrison Formation (Engelmann and Callison, 1998) are far removed from any living mammals in their history of adaptive modification. They are therefore of little value as environmental indicators. The specific adaptations of the Morrison mammals are not apparent from what is known of their anatomy. It seems likely that at least some, such as the triconodonts and dryolestoids, depended on a diet of small invertebrates and possibly small vertebrates. Whether some of the Morrison mammals, such as the multituberculates, may have been partly or completely herbivorous, cannot be determined with confidence. Thus, some, and possibly all of the Morrison mammals, as secondary consumers, were not immediately dependent on the vegetation for food. One characteristic common to the Morrison mammals is their small size. The largest were only the size of a modern ground squirrel, and many were much smaller. Because of their small size, the mammals probably would have been restricted to areas where water was continuously available. However, resource requirements for very small individual organisms are small, and a population of mammals could survive on very limited resources. Small animals also may be able to take advantage of microhabitats that offer more favorable conditions. For example, they may have been primarily nocturnal, sheltering in burrows to avoid the heat of the day. On the other hand, small size also implies short generation times, allowing surviving populations to expand rapidly when resources are relatively abundant [8]. --- Unique ecosystem The extinct ecosystem of the Morrison does appear to be unique, with no completely satisfactory modern analogue. One of its most peculiar features is the diverse fauna of sauropod dinosaurs. They undoubtedly had a central role in the ecosystem, and their presence and activities probably affected it in ways we have not considered or even imagined. However, we believe the scenario we have suggested resolves the information we have about the Morrison from diverse sources [8]. --- The Morrison invertebrates are instructive The invertebrate faunas in the Upper Jurassic Morrison Formation have received little attention in comparison to their better-known dinosaurian counterparts. Unlike the more prolifically preserved dinosaurian assemblages, invertebrates are rare as body fossils with some groups, like insects, being virtually absent from the fossil record (Gorman et al., 2008; Hasiotis, 2004; Lara et al., 2020; Zherikhin, 2003). Incorporating traces fossils, such as burrows, tracks, borings and feeding marks, has revealed a more complete picture of invertebrate diversity within Late Jurassic ecosystems in North America, and the ichnological record of invertebrate traces made on geological substrates has been documented from sites across the Morrison Formation (Hasiotis, 1999, 2004; Turner & Peterson, 2004). Among previous works examining invertebrate traces, those specifically left on vertebrate bone have received less targeted research [9]. Here, we present a robust dataset of invertebrate traces on vertebrate fossil material from the Upper Jurassic Mygatt-Moore Quarry (MMQ) in western Colorado [9]. The MMQ was discovered in 1981 by J.D. and Vanetta Moore and Pete and Marilyn Mygatt while hiking Rabbit Valley in the McInnis Canyons National Conservation Area, near the Utah-Colorado border (Fig. 1). The site preserves a dinosaur-dominated fossil assemblage within the Brushy Basin Member of the Upper Jurassic Morrison Formation [9]. Invertebrate faunas can also provide insights into paleoclimatic conditions in the fossil record (Elias, 2010; Guiot et al., 1993; Hasiotis, 2004; Holden et al., 2017). Data from experimental studies using dermestid and clerid beetles have demonstrated that these organisms prefer desiccated, though not wholly dry, vertebrate carcasses (Timm, 1982; Zanetti, Visciarelli & Centeno, 2014, 2015) and typically inhabit areas with temperatures above 20 degrees Celcius (Wilches et al., 2016). As dermestids, or behaviorally convergent taxa, are putative actors for the majority of this dataset, we can infer widespread desiccation among the modified remains and a warm, arid paleoclimate, consistent with previous interpretations of ecologically-stressed, resource-poor, drought-induced death assemblages in the Morrison Formation (Foster et al., 2018; Turner & Peterson, 2004) (Fig. 8). However, the presence of gastropods in the assemblage, as both body fossils and potential actors for Trace Type 3, reveals the fluctuation of the local climate at the MMQ and support the presence of periodic wet episodes. Additionally, sedimentological evidence of small (<1 cm2) interference ripple marks in the top 50 cm of the horizon and isolated pockets of bioturbation in the lowest meter of the horizon, presumably from dinosaurian faunas churning the smectitic muds like a boggy mire (Foster, 2003, 2014; Foster et al., 2018; McHugh, 2018; McHugh et al., 2018; Mygatt, 1991; Trujillo et al., 2014), support interpretations of the wet/dry seasonality at the MMQ. The disproportionate abundance of Trace Type 1 (dermestid or clerid beetles) compared to Trace Type 3 (gastropods or beetles) in the dataset indicates the dominance of dry conditions during the accumulation of vertebrate carcass remains at the site [9]. --- Two-thirds of biota represent just 1 Ma interval of existence? Two new chemical abrasion thermal ionization mass spectrometry U/Pb dates establish the age of the top of the Brushy Basin Member, Morrison Formation, at Flat Top (also known as Terrace Hill), north of the Fruita Paleontological Research Natural Area (FP), and of the lower part of the Brushy Basin Member, in the region of the Trail through Time (TT) and Mygatt-Moore Dinosaur Quarry in westernmost Colorado. Strata at both locations preserve a fossil record of large dinosaurs, including bones, dinosaur nests, and trace fossils, as well as fossils of unionid bivalves, fish, turtles, crocodilians, microvertebrates, logs, and other plant material. We report here a new weighted mean 206Pb/238U date of 149.43 +/- 0.059 Ma for three zircon grains sampled from a stratigraphic level 99 m above the base of a 101-m-thick Brushy Basin Member FP section and a new weighted mean 206Pb/238U date of 150.208 +/- 0.094 Ma for three zircon grains sampled from a stratigraphic level 39 m above the base of a 79-m-thick Brushy Basin Member TT section. These dates place the Brushy Basin strata in the Tithonian Stage of the Upper Jurassic Series. The 149.43 Ma date from Fruita is the youngest reliable date from near the top of the formation. The new dates also indicate a relatively short interval of deposition—less than 1 My—for the upper Brushy Basin Member. Thus, two-thirds of the dinosaur and other vertebrate fossils known from the Morrison Formation represent a biota that existed for only 1My. However, it is possible that some taxa persisted during some of the time represented by the K-1 unconformity [10]. | Saurophaganax maximus noticed in the Morrison Formation of Oklahoma [11]. Torvosaurus tanneri from the Morrison Formation in Colorado, Utah, and Wyoming [11]. Genus Allosaurus [11] Genus Ceratosaurus [11] |
Figures (1 - 2) in [8] for reference:

Figure 8 in [9] for reference:
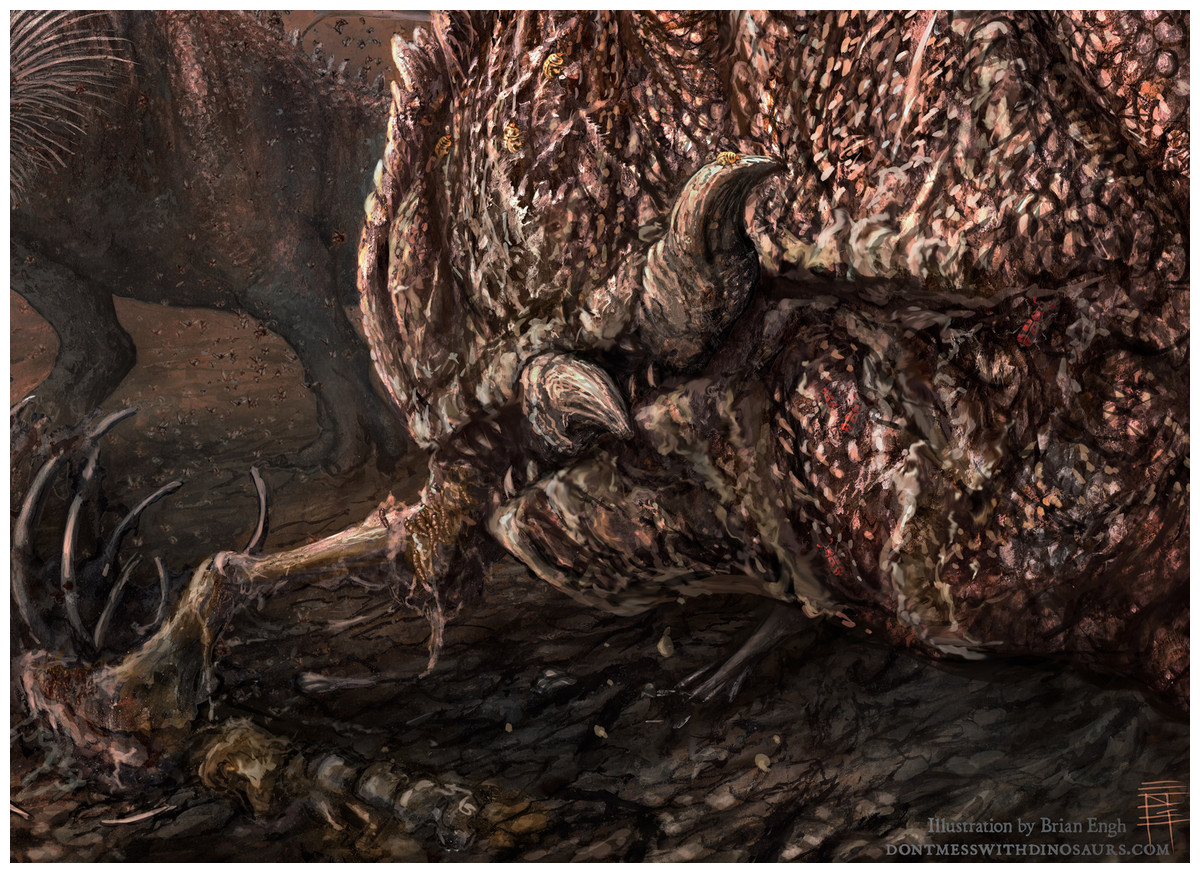
Figure 8: Artist Reconstruction.
Dry season at the Mygatt-Moore Quarry showing Allosaurus feeding on a rotting, bug-infested carcass. Illustration by Brian Engh, dontmesswithdinosaurs.com (modified with permission from Drumheller et al., 2020).
-----
REFERENCES
[1] LINK: www.climate.gov/news-features/climate-qa/whats-hottest-earths-ever-been
[2] LINK: organismalbio.biosci.gatech.edu/biodiversity/mass-extinctions-and-climate-variability-2/
[3] Bittencourt, J. S., & Langer, M. C. (2011). Mesozoic dinosaurs from Brazil and their biogeographic implications. Anais da Academia Brasileira de Ciências, 83(1), 23-60.
[4] Hudgins, M. N., Uhen, M. D., & Hinnov, L. A. (2020). The evolution of respiratory systems in Theropoda and Paracrocodylomorpha, the end-Triassic extinction, and the role of Late Triassic atmospheric O2 and CO2. Palaeogeography, Palaeoclimatology, Palaeoecology, 545, 109638.
[5] Adopted from the following link: www.geosociety.org/GSA/Education_Careers/Geologic_Time_Scale/GSA/timescale/home.aspx
[6] Holz, M. (2015). Mesozoic paleogeography and paleoclimates–a discussion of the diverse greenhouse and hothouse conditions of an alien world. Journal of South American Earth Sciences, 61, 91-107.
[7] Parrish, J. T., Peterson, F., & Turner, C. E. (2004). Jurassic “savannah”—plant taphonomy and climate of the Morrison Formation (Upper Jurassic, Western USA). Sedimentary Geology, 167(3-4), 137-162.
[8] Engelmann, G. F., Chure, D. J., & Fiorillo, A. R. (2004). The implications of a dry climate for the paleoecology of the fauna of the Upper Jurassic Morrison Formation. Sedimentary Geology, 167(3-4), 297-308.
[9] McHugh, J. B., Drumheller, S. K., Riedel, A., & Kane, M. (2020). Decomposition of dinosaurian remains inferred by invertebrate traces on vertebrate bone reveal new insights into Late Jurassic ecology, decay, and climate in western Colorado. PeerJ, 8, e9510.
[10] Galli, K. G., Buchwaldt, R., Lucas, S. G., & Tanner, L. (2018). New chemical abrasion thermal ionization mass spectrometry dates from the Brushy Basin Member, Morrison Formation, western Colorado: implications for dinosaur evolution. The Journal of Geology, 126(5), 473-486.
[11] Drumheller, S. K., McHugh, J. B., Kane, M., Riedel, A., & D’Amore, D. C. (2020). High frequencies of theropod bite marks provide evidence for feeding, scavenging, and possible cannibalism in a stressed Late Jurassic ecosystem. Plos one, 15(5), e0233115.