Post by Runic on Aug 28, 2013 22:15:14 GMT 5
DEINONYCHUS ANTIRROPHUS
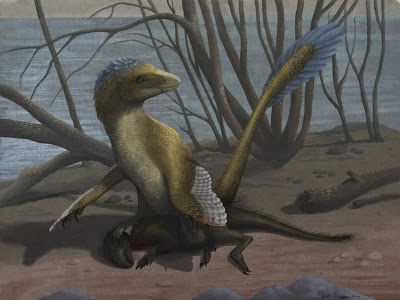
Phylum: Chordata
Clade: Avemetatarsalia
Family: †Dromaeosauridae
Clade: †Eudromaeosauria
Subfamily: †Velociraptorinae
Genus: †Deinonychus
Ostrom, 1969
Species: † D. antirrhopus
Deinonychus (/da??n?n?k?s/ dy-non-i-k?s; Greek:
, 'terrible' and
?, genitive 
'claw') is a genus of carnivorous dromaeosaurid dinosaurs. There is one described species, Deinonychus antirrhopus. These dinosaurs, which could grow up to 3.4 meters (11 ft) long, lived during the early Cretaceous Period, about 115–108 million years ago (from the mid-Aptian to early Albian stages). Fossils have been recovered from the U.S. states of Montana, Wyoming, and Oklahoma, in rocks of the Cloverly Formation and Antlers Formation, though teeth that may belong to Deinonychus have been found much farther east in Maryland.
Paleontologist John Ostrom's study of Deinonychus in the late 1960s revolutionized the way scientists thought about dinosaurs, leading to the "dinosaur renaissance" and igniting the debate on whether dinosaurs were warm-blooded or cold blooded. Before this, the popular conception of dinosaurs had been one of plodding, reptilian giants. Ostrom noted the small body, sleek, horizontal posture, ratite-like spine, and especially the enlarged raptorial claws on the feet, which suggested an active, agile predator.[1]
"Terrible claw" refers to the unusually large, sickle-shaped talon on the second toe of each hind foot. The fossil YPM 5205 preserves a large, strongly curved ungual. In life, archosaurs have a horny sheath over this bone, which extends the length. Ostrom looked at crocodile and bird claws and reconstructed the claw for YPM 5205 as over 120 millimetres (4.7 in) long.[1] The species name antirrhopus means "counter balance", which refers to Ostrom's idea about the function of the tail. As in other dromaeosaurids, the tail vertebrae have a series of ossified tendons and super-elongated bone processes. These features seemed to make the tail into a stiff counterbalance, but a fossil of the very closely related Velociraptor mongoliensis (IGM 100/986) has an articulated tail skeleton that is curved laterally in a long S–shape. This suggests that, in life, the tail could bend to the sides with a high degree of flexibility.[2]
In both the Cloverly and Antlers formations, Deinonychus remains have been found closely associated with those of the ornithopod Tenontosaurus. Teeth discovered associated with Tenontosaurus specimens imply they were hunted, or at least scavenged upon, by Deinonychus.
Based on the largest known specimens, Deinonychus could reach at least 3.4 metres (11 ft 2 in) in length, with a skull length of 410 mm (16.1 in), a hip height of 0.87 metres (2 ft 10 in), and an estimated weight of 73 kilograms (160 lb).[3] The skull was equipped with powerful jaws lined with around seventy curved, blade-like teeth. Studies of the skull have progressed a great deal over the decades. Ostrom reconstructed the partial, imperfectly preserved skulls that he had as triangular, broad, and fairly similar to Allosaurus. Additional Deinonychus skull material and closely related species found with good three-dimensional preservation[4] show that the palate was more vaulted than Ostrom thought, making the snout far narrower, while the jugals flared broadly, giving greater stereoscopic vision. The skull of Deinonychus was different from that of Velociraptor, however, in that it had a more robust skull roof, like that of Dromaeosaurus, and did not have the depressed nasals of Velociraptor.[5] Both the skull and the lower jaw had fenestrae (skull openings) which reduced the weight of the skull. In Deinonychus, the antorbital fenestra, a skull opening between the eye and nostril, was particularly large.[4]
Deinonychus possessed large "hands" (manus) with three claws on each forelimb. The first digit was shortest and the second was longest. Each hind foot bore a sickle-shaped claw on the second digit, which was probably used during predation.[6]
No skin impressions have ever been found in association with fossils of Deinonychus. Nonetheless, the evidence suggests that the Dromaeosauridae, including Deinonychus, had feathers.[7] The genus Microraptor is both older geologically and more primitive phylogenetically than Deinonychus, and within the same family.[8] Multiple fossils of Microraptor preserve pennaceous, vaned feathers like those of modern birds on the arms, legs, and tail, along with covert and contour feathers.[7] Velociraptor is geologically younger than Deinonychus, but even more closely related (within the subfamily velociraptorinae, see Classification, below). A specimen of Velociraptor has been found with quill knobs on the ulna. Quill knobs are where the follicular ligaments attached, and are a direct indicator of feathers of modern aspect.[9]
Fossilized remains of Deinonychus have been recovered from the Cloverly Formation of Montana and Wyoming[1] and in the roughly contemporary Antlers Formation of Oklahoma,[17] in North America. The Cloverly formation has been dated to the late Aptian through early Albian stages of the early Cretaceous, about 115 to 108 Ma.[18][19] Additionally, teeth found in the Arundel Clay Facies (mid-Aptian), of the Potomac Formation on the Atlantic Coastal Plain of Maryland may be assigned to the genus.[20]
The first remains were uncovered in 1931 in southern Montana near the town of Billings. The team leader, paleontologist Barnum Brown, was primarily concerned with excavating and preparing the remains of the ornithopod dinosaur Tenontosaurus, but in his field report from the dig site to the American Museum of Natural History, he reported the discovery of a small carnivorous dinosaur close to a Tenontosaurus skeleton, "but encased in lime difficult to prepare."[21] He informally called the animal "Daptosaurus agilis" and made preparations for describing it and having the skeleton, specimen AMNH 3015, put on display, but never finished this work.[22] Brown brought back from the Cloverly Formation the skeleton of a smaller theropod with seemingly oversized teeth that he informally named "Megadontosaurus". John Ostrom, reviewing this material decades later, realized that the teeth came from Deinonychus, but the skeleton came from a completely different animal. He named this skeleton Microvenator.[22]
A little more than thirty years later, in August 1964, paleontologist John Ostrom led an expedition from Yale University's Peabody Museum which discovered more skeletal material near Bridger. Expeditions during the following two summers uncovered more than 1,000 bones, among which were at least three individuals. Since the association between the various recovered bones was weak, making the exact number of individual animals represented impossible to determine properly, the type specimen (YPM 5205) of Deinonychus was restricted to the complete left foot and partial right foot that definitely belonged to the same individual.[6] The remaining specimens were catalogued in fifty separate entries at Yale's Peabody Museum of Natural History.
Cast of the holotype foot YPM 5205

Later study by Ostrom and Grant E. Meyer analyzed their own material as well as Brown's "Daptosaurus" in detail and found them to be the same species. Ostrom first published his findings in February 1969, giving all the referred remains the new name of Deinonychus antirrhopus. The specific name "antirrhopus", from Greek

?, means "counterbalancing" and refers to the likely purpose of a stiffened tail.[23] In July 1969, Ostrom published a very extensive monograph on Deinonychus.[6]
Though a myriad of bones was available by 1969, many important ones were missing or hard to interpret. There were few postorbital skull elements, no femurs, no sacrum, no furcula or sternum, missing vertebrae, and (Ostrom thought) only a tiny fragment of a coracoid. Ostrom's skeletal reconstruction of Deinonychus included a very unusual pelvic bone – a pubis that was trapezoidal and flat, unlike that of other theropods, but which was the same length as the ischium and which was found right next to it.
Further findings
In 1974, Ostrom published another monograph on the shoulder of Deinonychus in which he realized that the pubis that he had described was actually a coracoid – a shoulder element.[24] In that same year, another specimen of Deinonychus, MCZ 4371, was discovered and excavated in Montana by Steven Orzack during a Harvard University expedition headed by Farish Jenkins. This discovery added several new elements: well preserved femurs, pubes, a sacrum, and better ilia, as well as elements of the pes and metatarsus. Ostrom described this specimen and revised his skeletal restoration of Deinonychus. This time it showed the very long pubis, and Ostrom began to suspect that they may have even been a little retroverted like those of birds.[25]
A skeleton of Deinonychus, including bones from the original (and most complete) AMNH 3015 specimen, can be seen on display at the American Museum of Natural History,[26] with another specimen (MCZ 4371) on display at the Museum of Comparative Zoology at Harvard University. The American Museum and Harvard specimens are from a different locality than the Yale specimens. Even these two skeletal mounts are lacking elements, including the sterna, sternal ribs, furcula, and gastralia.
Even after all of Ostrom's work, several small blocks of lime-encased material remained unprepared in storage at the American Museum. These consisted mostly of isolated bones and bone fragments, including the original matrix, or surrounding rock in which the specimens were initially buried. An examination of these unprepared blocks by Gerald Grellet-Tinner and Peter Makovicky in 2000 revealed an interesting, overlooked feature. Several long, thin bones identified on the blocks as ossified tendons (structures that helped stiffen the tail of Deinonychus) turned out to actually represent gastralia (abdominal ribs). More significantly, a large number of previously unnoticed fossilized eggshells were discovered in the rock matrix that had surrounded the original Deinonychus specimen.[27]
In a subsequent, more detailed report on the eggshells, Grellet-Tinner and Makovicky concluded that the egg almost certainly belonged to Deinonychus, representing the first dromaeosaurid egg to be identified.[21] Moreover, the external surface of one eggshell was found in close contact with the gastralia suggesting that Deinonychus might have brooded its eggs. This implies that Deinonychus used body heat transfer as a mechanism for egg incubation, and indicates an endothermy similar to modern birds.[28] Further study by Gregory Erickson and colleagues finds that this individual was 13 or 14 years old at death and its growth had plateaued. Unlike other theropods in their study of specimens found associated with eggs or nests, it had finished growing at the time of its death.[29]
Implications
The similarity of the forelimbs (left) with those of Archaeopteryx (right) led John Ostrom to revive the link between dinosaurs and birds
Ostrom's description of Deinonychus in 1969 has been described as the most important single discovery of dinosaur paleontology in the mid-20th century.[30] The discovery of this clearly active, agile predator did much to change the scientific (and popular) conception of dinosaurs and opened the door to speculation that dinosaurs may have been warm-blooded. This development has been termed the dinosaur renaissance. Several years later, Ostrom noted similarities between the forefeet of Deinonychus and that of birds, an observation which led him to revive the hypothesis that birds are descended from dinosaurs.[31] Forty years later, this idea is almost universally accepted.
Because of its extremely bird-like anatomy and close relationship to other dromaeosaurids, paleontologists hypothesize that Deinonychus was probably covered in feathers.[32][33][34] Clear fossil evidence of modern avian-style feathers exists for several related dromaeosaurids, including Velociraptor and Microraptor, though no direct evidence is yet known for Deinonychus itself.[7][9] When conducting studies of such areas as the range of motion in the forelimbs, paleontologists like Phil Senter have taken the likely presence of wing feathers (as present in all known dromaeosaurs with skin impressions) into consideration.[35]
Deinonychus teeth found in association with fossils of the ornithopod dinosaur Tenontosaurus are quite common in the Cloverly Formation. Two quarries have been discovered that preserve fairly complete Deinonychus fossils near Tenontosaurus fossils. The first, the Yale quarry in the Cloverly of Montana, includes numerous teeth, four adult Deinonychus and one juvenile Deinonychus. The association of this number of Deinonychus skeletons in a single quarry suggests that Deinonychus may have fed on that animal, and perhaps hunted it. Ostrom and Maxwell have even used this information to speculate that Deinonychus might have lived and hunted in packs.[39] The second such quarry is from the Antlers Formation of Oklahoma. The site contains six partial skeletons of Tenontosaurus of various sizes, along with one partial skeleton and many teeth of Deinonychus. One tenontosaur humerus even bears what might be Deinonychus tooth marks. Brinkman et al. (1998) point out that Deinonychus had an adult mass of 70–100 kilograms, whereas adult tenontosaurs were 1–4 metric tons. A solitary Deinonychus could not kill an adult tenontosaur, suggesting that pack hunting is possible.[17]
A 2007 study by Roach and Brinkman has called into question the cooperative pack hunting behavior of Deinonychus, based on what is known of modern carnivore hunting and the taphonomy of tenontosaur sites. Modern archosaurs (birds and crocodiles) and komodo dragons display little cooperative hunting; instead, they are usually either solitary hunters, or are drawn to previously killed carcasses, where much conflict occurs between individuals of the same species. For example, in situations where groups of komodo dragons are eating together, the largest individuals eat first and will attack smaller komodos that attempt to feed; if the smaller animal is killed, it is cannibalized. When this information is applied to the tenontosaur sites, it appears that what is found is consistent with Deinonychus having a komodo- or crocodile-like feeding strategy. Deinonychus skeletal remains found at these sites are from subadults, with missing parts consistent with having been eaten by other Deinonychus.[40] On the other hand, a paper by Li et al. describes track sites with similar foot spacing and parallel trackways, implying gregarious packing behavior instead of uncoordinated feeding behavior.[41]
In 2011, Denver Fowler and colleagues suggested a new method by which Deinonychus and other dromaeosaurs may have captured and restrained prey.[42] This model, known as the "raptor prey restraint" (RPR) model of predation, proposes that Deinonychus killed its prey in a manner very similar to extant accipitrid birds of prey: by leaping onto its quarry, pinning it under its body weight, and gripping it tightly with the large, sickle-shaped claws. Like accipitrids, the dromaeosaur would then begin to feed on the animal while still alive, until it eventually died from blood loss and organ failure. This proposal is based primarily on comparisons between the morphology and proportions of the feet and legs of dromaeosaurs to several groups of extant birds of prey with known predatory behaviors. Fowler found that the feet and legs of dromaeosaurs most closely resemble those of eagles and hawks, especially in terms of having an enlarged second claw and a similar range of grasping motion. However, the short metatarsus and foot strength would have been more similar to that of owls. The RPR method of predation would be consistent with other aspects of Deinonychus's anatomy, such as their unusual jaw and arm morphology. The arms were likely covered in long feathers, and may have been used as flapping stabilizers for balance while atop a struggling prey animal, along with the stiff counterbalancing tail. The jaws, thought to have had a comparatively weak bite force,[43] would have been useful for eating prey alive but not as useful for quick, forceful dispatch of the prey (since modern animals that do this have unique specializations that dromaeosaurs lacked). These predatory adaptations working together may also have implications for the origin of flapping in paravians.
Bite force estimates for Deinonychus were first produced in 2005, based on reconstructed jaw musculature. This study concluded that Deinonychus likely had a maximum bite force only 15% that of the modern American Alligator.[43] A 2010 study by Paul Gignac and colleagues attempted to estimate the bite force based directly on newly discovered Deinonychus tooth puncture marks in the bones of a Tenontosaurus. These puncture marks came from a large individual, and provided the first evidence that large Deinonychus could bite through bone. Using the tooth marks, Gignac's team were able to determine that the bite force of Deinonychus was significantly higher than earlier studies had estimated by biomechanical studies alone. They found the bite force of Deinonychus to be between 4,100 and 8,200 newtons, greater than living carnivorous mammals including the hyena, and equivalent to a similarly sized alligator.
Gignac and colleagues also noted, however, that bone puncture marks from Deinonychus are relatively rare, and unlike larger theropods with many known puncture marks like Tyrannosaurus, Deinonychus probably did not frequently bite through or eat bone. Instead, they probably used their high bite force in defense or prey capture, rather than feeding.[44]
My post from Carnivoraforum below on Deinonychus and dromaeosaurid grappling abilities
---------
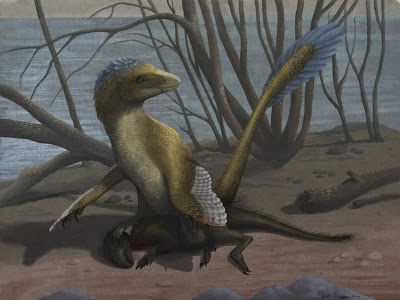
Phylum: Chordata
Clade: Avemetatarsalia
Family: †Dromaeosauridae
Clade: †Eudromaeosauria
Subfamily: †Velociraptorinae
Genus: †Deinonychus
Ostrom, 1969
Species: † D. antirrhopus
Deinonychus (/da??n?n?k?s/ dy-non-i-k?s; Greek:





Paleontologist John Ostrom's study of Deinonychus in the late 1960s revolutionized the way scientists thought about dinosaurs, leading to the "dinosaur renaissance" and igniting the debate on whether dinosaurs were warm-blooded or cold blooded. Before this, the popular conception of dinosaurs had been one of plodding, reptilian giants. Ostrom noted the small body, sleek, horizontal posture, ratite-like spine, and especially the enlarged raptorial claws on the feet, which suggested an active, agile predator.[1]
"Terrible claw" refers to the unusually large, sickle-shaped talon on the second toe of each hind foot. The fossil YPM 5205 preserves a large, strongly curved ungual. In life, archosaurs have a horny sheath over this bone, which extends the length. Ostrom looked at crocodile and bird claws and reconstructed the claw for YPM 5205 as over 120 millimetres (4.7 in) long.[1] The species name antirrhopus means "counter balance", which refers to Ostrom's idea about the function of the tail. As in other dromaeosaurids, the tail vertebrae have a series of ossified tendons and super-elongated bone processes. These features seemed to make the tail into a stiff counterbalance, but a fossil of the very closely related Velociraptor mongoliensis (IGM 100/986) has an articulated tail skeleton that is curved laterally in a long S–shape. This suggests that, in life, the tail could bend to the sides with a high degree of flexibility.[2]
In both the Cloverly and Antlers formations, Deinonychus remains have been found closely associated with those of the ornithopod Tenontosaurus. Teeth discovered associated with Tenontosaurus specimens imply they were hunted, or at least scavenged upon, by Deinonychus.
Based on the largest known specimens, Deinonychus could reach at least 3.4 metres (11 ft 2 in) in length, with a skull length of 410 mm (16.1 in), a hip height of 0.87 metres (2 ft 10 in), and an estimated weight of 73 kilograms (160 lb).[3] The skull was equipped with powerful jaws lined with around seventy curved, blade-like teeth. Studies of the skull have progressed a great deal over the decades. Ostrom reconstructed the partial, imperfectly preserved skulls that he had as triangular, broad, and fairly similar to Allosaurus. Additional Deinonychus skull material and closely related species found with good three-dimensional preservation[4] show that the palate was more vaulted than Ostrom thought, making the snout far narrower, while the jugals flared broadly, giving greater stereoscopic vision. The skull of Deinonychus was different from that of Velociraptor, however, in that it had a more robust skull roof, like that of Dromaeosaurus, and did not have the depressed nasals of Velociraptor.[5] Both the skull and the lower jaw had fenestrae (skull openings) which reduced the weight of the skull. In Deinonychus, the antorbital fenestra, a skull opening between the eye and nostril, was particularly large.[4]
Deinonychus possessed large "hands" (manus) with three claws on each forelimb. The first digit was shortest and the second was longest. Each hind foot bore a sickle-shaped claw on the second digit, which was probably used during predation.[6]
No skin impressions have ever been found in association with fossils of Deinonychus. Nonetheless, the evidence suggests that the Dromaeosauridae, including Deinonychus, had feathers.[7] The genus Microraptor is both older geologically and more primitive phylogenetically than Deinonychus, and within the same family.[8] Multiple fossils of Microraptor preserve pennaceous, vaned feathers like those of modern birds on the arms, legs, and tail, along with covert and contour feathers.[7] Velociraptor is geologically younger than Deinonychus, but even more closely related (within the subfamily velociraptorinae, see Classification, below). A specimen of Velociraptor has been found with quill knobs on the ulna. Quill knobs are where the follicular ligaments attached, and are a direct indicator of feathers of modern aspect.[9]
Fossilized remains of Deinonychus have been recovered from the Cloverly Formation of Montana and Wyoming[1] and in the roughly contemporary Antlers Formation of Oklahoma,[17] in North America. The Cloverly formation has been dated to the late Aptian through early Albian stages of the early Cretaceous, about 115 to 108 Ma.[18][19] Additionally, teeth found in the Arundel Clay Facies (mid-Aptian), of the Potomac Formation on the Atlantic Coastal Plain of Maryland may be assigned to the genus.[20]
The first remains were uncovered in 1931 in southern Montana near the town of Billings. The team leader, paleontologist Barnum Brown, was primarily concerned with excavating and preparing the remains of the ornithopod dinosaur Tenontosaurus, but in his field report from the dig site to the American Museum of Natural History, he reported the discovery of a small carnivorous dinosaur close to a Tenontosaurus skeleton, "but encased in lime difficult to prepare."[21] He informally called the animal "Daptosaurus agilis" and made preparations for describing it and having the skeleton, specimen AMNH 3015, put on display, but never finished this work.[22] Brown brought back from the Cloverly Formation the skeleton of a smaller theropod with seemingly oversized teeth that he informally named "Megadontosaurus". John Ostrom, reviewing this material decades later, realized that the teeth came from Deinonychus, but the skeleton came from a completely different animal. He named this skeleton Microvenator.[22]
A little more than thirty years later, in August 1964, paleontologist John Ostrom led an expedition from Yale University's Peabody Museum which discovered more skeletal material near Bridger. Expeditions during the following two summers uncovered more than 1,000 bones, among which were at least three individuals. Since the association between the various recovered bones was weak, making the exact number of individual animals represented impossible to determine properly, the type specimen (YPM 5205) of Deinonychus was restricted to the complete left foot and partial right foot that definitely belonged to the same individual.[6] The remaining specimens were catalogued in fifty separate entries at Yale's Peabody Museum of Natural History.
Cast of the holotype foot YPM 5205

Later study by Ostrom and Grant E. Meyer analyzed their own material as well as Brown's "Daptosaurus" in detail and found them to be the same species. Ostrom first published his findings in February 1969, giving all the referred remains the new name of Deinonychus antirrhopus. The specific name "antirrhopus", from Greek



Though a myriad of bones was available by 1969, many important ones were missing or hard to interpret. There were few postorbital skull elements, no femurs, no sacrum, no furcula or sternum, missing vertebrae, and (Ostrom thought) only a tiny fragment of a coracoid. Ostrom's skeletal reconstruction of Deinonychus included a very unusual pelvic bone – a pubis that was trapezoidal and flat, unlike that of other theropods, but which was the same length as the ischium and which was found right next to it.
Further findings
In 1974, Ostrom published another monograph on the shoulder of Deinonychus in which he realized that the pubis that he had described was actually a coracoid – a shoulder element.[24] In that same year, another specimen of Deinonychus, MCZ 4371, was discovered and excavated in Montana by Steven Orzack during a Harvard University expedition headed by Farish Jenkins. This discovery added several new elements: well preserved femurs, pubes, a sacrum, and better ilia, as well as elements of the pes and metatarsus. Ostrom described this specimen and revised his skeletal restoration of Deinonychus. This time it showed the very long pubis, and Ostrom began to suspect that they may have even been a little retroverted like those of birds.[25]
A skeleton of Deinonychus, including bones from the original (and most complete) AMNH 3015 specimen, can be seen on display at the American Museum of Natural History,[26] with another specimen (MCZ 4371) on display at the Museum of Comparative Zoology at Harvard University. The American Museum and Harvard specimens are from a different locality than the Yale specimens. Even these two skeletal mounts are lacking elements, including the sterna, sternal ribs, furcula, and gastralia.
Even after all of Ostrom's work, several small blocks of lime-encased material remained unprepared in storage at the American Museum. These consisted mostly of isolated bones and bone fragments, including the original matrix, or surrounding rock in which the specimens were initially buried. An examination of these unprepared blocks by Gerald Grellet-Tinner and Peter Makovicky in 2000 revealed an interesting, overlooked feature. Several long, thin bones identified on the blocks as ossified tendons (structures that helped stiffen the tail of Deinonychus) turned out to actually represent gastralia (abdominal ribs). More significantly, a large number of previously unnoticed fossilized eggshells were discovered in the rock matrix that had surrounded the original Deinonychus specimen.[27]
In a subsequent, more detailed report on the eggshells, Grellet-Tinner and Makovicky concluded that the egg almost certainly belonged to Deinonychus, representing the first dromaeosaurid egg to be identified.[21] Moreover, the external surface of one eggshell was found in close contact with the gastralia suggesting that Deinonychus might have brooded its eggs. This implies that Deinonychus used body heat transfer as a mechanism for egg incubation, and indicates an endothermy similar to modern birds.[28] Further study by Gregory Erickson and colleagues finds that this individual was 13 or 14 years old at death and its growth had plateaued. Unlike other theropods in their study of specimens found associated with eggs or nests, it had finished growing at the time of its death.[29]
Implications
The similarity of the forelimbs (left) with those of Archaeopteryx (right) led John Ostrom to revive the link between dinosaurs and birds
Ostrom's description of Deinonychus in 1969 has been described as the most important single discovery of dinosaur paleontology in the mid-20th century.[30] The discovery of this clearly active, agile predator did much to change the scientific (and popular) conception of dinosaurs and opened the door to speculation that dinosaurs may have been warm-blooded. This development has been termed the dinosaur renaissance. Several years later, Ostrom noted similarities between the forefeet of Deinonychus and that of birds, an observation which led him to revive the hypothesis that birds are descended from dinosaurs.[31] Forty years later, this idea is almost universally accepted.
Because of its extremely bird-like anatomy and close relationship to other dromaeosaurids, paleontologists hypothesize that Deinonychus was probably covered in feathers.[32][33][34] Clear fossil evidence of modern avian-style feathers exists for several related dromaeosaurids, including Velociraptor and Microraptor, though no direct evidence is yet known for Deinonychus itself.[7][9] When conducting studies of such areas as the range of motion in the forelimbs, paleontologists like Phil Senter have taken the likely presence of wing feathers (as present in all known dromaeosaurs with skin impressions) into consideration.[35]
Deinonychus teeth found in association with fossils of the ornithopod dinosaur Tenontosaurus are quite common in the Cloverly Formation. Two quarries have been discovered that preserve fairly complete Deinonychus fossils near Tenontosaurus fossils. The first, the Yale quarry in the Cloverly of Montana, includes numerous teeth, four adult Deinonychus and one juvenile Deinonychus. The association of this number of Deinonychus skeletons in a single quarry suggests that Deinonychus may have fed on that animal, and perhaps hunted it. Ostrom and Maxwell have even used this information to speculate that Deinonychus might have lived and hunted in packs.[39] The second such quarry is from the Antlers Formation of Oklahoma. The site contains six partial skeletons of Tenontosaurus of various sizes, along with one partial skeleton and many teeth of Deinonychus. One tenontosaur humerus even bears what might be Deinonychus tooth marks. Brinkman et al. (1998) point out that Deinonychus had an adult mass of 70–100 kilograms, whereas adult tenontosaurs were 1–4 metric tons. A solitary Deinonychus could not kill an adult tenontosaur, suggesting that pack hunting is possible.[17]
A 2007 study by Roach and Brinkman has called into question the cooperative pack hunting behavior of Deinonychus, based on what is known of modern carnivore hunting and the taphonomy of tenontosaur sites. Modern archosaurs (birds and crocodiles) and komodo dragons display little cooperative hunting; instead, they are usually either solitary hunters, or are drawn to previously killed carcasses, where much conflict occurs between individuals of the same species. For example, in situations where groups of komodo dragons are eating together, the largest individuals eat first and will attack smaller komodos that attempt to feed; if the smaller animal is killed, it is cannibalized. When this information is applied to the tenontosaur sites, it appears that what is found is consistent with Deinonychus having a komodo- or crocodile-like feeding strategy. Deinonychus skeletal remains found at these sites are from subadults, with missing parts consistent with having been eaten by other Deinonychus.[40] On the other hand, a paper by Li et al. describes track sites with similar foot spacing and parallel trackways, implying gregarious packing behavior instead of uncoordinated feeding behavior.[41]
In 2011, Denver Fowler and colleagues suggested a new method by which Deinonychus and other dromaeosaurs may have captured and restrained prey.[42] This model, known as the "raptor prey restraint" (RPR) model of predation, proposes that Deinonychus killed its prey in a manner very similar to extant accipitrid birds of prey: by leaping onto its quarry, pinning it under its body weight, and gripping it tightly with the large, sickle-shaped claws. Like accipitrids, the dromaeosaur would then begin to feed on the animal while still alive, until it eventually died from blood loss and organ failure. This proposal is based primarily on comparisons between the morphology and proportions of the feet and legs of dromaeosaurs to several groups of extant birds of prey with known predatory behaviors. Fowler found that the feet and legs of dromaeosaurs most closely resemble those of eagles and hawks, especially in terms of having an enlarged second claw and a similar range of grasping motion. However, the short metatarsus and foot strength would have been more similar to that of owls. The RPR method of predation would be consistent with other aspects of Deinonychus's anatomy, such as their unusual jaw and arm morphology. The arms were likely covered in long feathers, and may have been used as flapping stabilizers for balance while atop a struggling prey animal, along with the stiff counterbalancing tail. The jaws, thought to have had a comparatively weak bite force,[43] would have been useful for eating prey alive but not as useful for quick, forceful dispatch of the prey (since modern animals that do this have unique specializations that dromaeosaurs lacked). These predatory adaptations working together may also have implications for the origin of flapping in paravians.
Bite force estimates for Deinonychus were first produced in 2005, based on reconstructed jaw musculature. This study concluded that Deinonychus likely had a maximum bite force only 15% that of the modern American Alligator.[43] A 2010 study by Paul Gignac and colleagues attempted to estimate the bite force based directly on newly discovered Deinonychus tooth puncture marks in the bones of a Tenontosaurus. These puncture marks came from a large individual, and provided the first evidence that large Deinonychus could bite through bone. Using the tooth marks, Gignac's team were able to determine that the bite force of Deinonychus was significantly higher than earlier studies had estimated by biomechanical studies alone. They found the bite force of Deinonychus to be between 4,100 and 8,200 newtons, greater than living carnivorous mammals including the hyena, and equivalent to a similarly sized alligator.
Gignac and colleagues also noted, however, that bone puncture marks from Deinonychus are relatively rare, and unlike larger theropods with many known puncture marks like Tyrannosaurus, Deinonychus probably did not frequently bite through or eat bone. Instead, they probably used their high bite force in defense or prey capture, rather than feeding.[44]
My post from Carnivoraforum below on Deinonychus and dromaeosaurid grappling abilities
---------
Yep forever finding more, This was a quote off how velociraptor form JP3 hands were horribly in the wrong position.
Jurassic Park Velociraptors are named after Velociraptor mongoliensis, and modeled after a hybrid between the genera Deinonychus and Utahraptor. At the time the novel was written, there was some talk of moving Deinonychus into the genus Velociraptor, which was the convention followed by Micheal Crichton, though this didn't play out. However, all of these were dromaeosaurids, which are eumaniraptorans. In fact, Dromaeosauridae is the group closest to but outside of Aves. So, all members of eumaniraptora have a synapomorphy list that includes a "semilunate carpal".



All three of the pictures above show this semilunate carpal in other maniraptorans. Unlike most mammalian wrists, it cannot flex perpendicular to the radius-ulna plane (ie, what we would most commonly accept as perpendicular to the groud). Instead, its motions were restricted to that plane, giving a jacknifing motion, much like a flight stroke. This was an optimal grasping motion for prey, and good for grappling large prey, and was easy to adapt into a flight stroke (particularly given the presence of primary feathers on some dromaeosaurids).
Sadly, this does mean that the raptors couldn't open doors. They would have broken their wrists trying to make the motion!
With Arms Wide Open (and Apologies to Pearl Jam)
I am very curious where this study will go next. Fowler et al. have produced a wealth of quanitified data regarding toe function and proportions (Fowler et al., 2009, 2011). These analyses attempt to place into a behavioral context the function of the dromaeosaur pes and thus feeding ecology on the whole. They took the “raptors” off the sides of the tenontosaurs and engaged them with smaller prey. Cautiously, then, one must note that this is all itself speculative. Indeed, the presence of large, flexible hands and apparent wings are themselves bizarrely-linked to the question of pedal function, as Fowler et al., 2011, themselves note:
It remains paradoxical that the manual digits of paravians seem well-suited for flexion and grasping, yet would have borne flight feathers so as to make such an action difficult or clumsy (Ostrom, 1969; Senter, 2006; Carpenter, 2002). Further, paravian manual unguals are enlarged and strong expression of flexor tubercles suggests that the claws were capable of exerting considerable force, yet the limited range of motion of the forelimb (Senter, 2006) seems strongly adapted for flapping, rather than the flexibility required for prey manipulation (or indeed, climbing (Dececchi & Larsson, 2011)).
[Fowler et al., 2011:pg.8-9; I've modified the internal reference numbers to citations, a practice of PLoS ONE's that makes internal text more legible, but reference checking more cumbersome. All cited references are included below.]
Fowler et al., 2011, primarily concern themselves with the “mid-sized” dromaeosaurids, Velociraptor mongoliensis and Deinonychus antirrhopus, but it should be noted that in the remarks above, most of the work has been to compare broadly the small nonavian theropods Microraptor zhaoianus (Dececchi & Larsson, 2011 [n2]) and Archaeopteryx lithographica (Ostrom, 1969). These analysis draw parallels based solely on the apparent articulation of the shoulder girdle, or later the apparent presence of a series of quill knobs on the particularly short ulna of Velociraptor mongoliensis (Turner et al., 2007b). While the articulation of the various elements of the shoulder are questionable (for example, check out Jason Brougham’s deconstruction of the topic at his blog, Soft Dinosaurs (and here are links to part one, two, three, four and five of this series, and an outlier discussion on dromaeosaur girdles in general, prompted by Burnham et al.’s (2000) improbably shoulder articulation for Bambiraptor feinbergi), absolute limb length implies that any mechanical leverage of the limbs and its range of motion was less than that of any extant volant-capable bird (Ostrom, 1969; Chiappe & Padian, 1998; Dial, 2003), although it was clearly greater than antecedents. It is also generally considered that Velociraptor mongoliensis and Deinonychus antirrhopus were terrestrial and non-volant in almost any fashion, yet Fowler et al., 2011, supposed they might have employed the flapping wingstroke in a form of stability flapping while engaged with prey. Thus regardless of their archaic (relative to extant birds) limb design and small pectoral and brachial muscle systems compared to, say Confuciusornis sanctus (Dececchi & Larsson, 2011), the “wings” of larger dromaeosaurids (as supposed by Fowler et al., 2011) are modeled directly after the smaller, older, and phylogenentically more basal deinonychosaurs, such as Archaeopteryx lithographica.
So what to make of the “flapping first” model versus the competing ideas? It is a compelling idea, and it takes the potential of integrating actual feeding ecology rather than circumstantial prey acquisition (such as a lizard, mammal or bird in the stomach or gut region) based on biomechanics into account. I much prefer this method of reasoning, and do not see any distinct problems with this especially in connection with WAIR (Wing-Assisted Incline Running, as proposed by Dial, 2003). It should be noted, however, that speculations on this stem directly from the largest of dromaeosaurs, not the smallest. It seems unreasonable to paint the short armed, robust and relatively shorter legged, probable ambush predators of the Djadokhta and Cloverly Formations in the same manner as the smaller “near-avians” of the Solnhofen, Yixian and Jiufotang Formations. Taking phylogenetics into account, dromaeosaurids became larger, less arboreal, as they evolved, rather than smaller and more arboreal, or more flight-capable. This implies that models based on the largest are ill-suited to help explain the smallest, or that the model can explain the unusual pedal integument of Microraptor zhaoianus, Anchiornis huxleyi or Archaeopteryx lithographica (Xu et al., 2003; Hu et al., 2009; Longrich, 2006).
These issues are actually complicated by the problems of incorporated a particularly grasping suited manus with a grasping suited pes, but relegate the actual grasping duty only to the pes, leaving the manus free. This should, on the path toward flapping or corralling behavior, lead to a stiffer manus (one of those “Just So Stories” we’ve been taught), yet the larger dromaeosaurs seem to have very flexible digits, especially the third manus digit (Senter, 2009). Moreover, it seems improbable that the arm was as winged as that of much smaller dromaeosaurs, where the wings are not broad and rounded but slender and tapering sharply (in contrast to accipiters). The tail, acting as a counter-balancing rod, seems odd in that in Microraptor gui, unlike Archaeopteryx lithographica (see Xu et al., 2003; Longrich, 2006) only the distal tip of the tail bore a “retricial” array. Absence of a distinct tail fan in raptors (any of them) implies that the distal end only structure may have had less of an effect during mantling when used to “corral” prey, than to cover it during invasive aggression of possible thieves, or threat displays. That this behavior (as Fowler et al., 2011, note) occurs when the prey is dead, the effect of maintaining purchase during the struggle may be less of an explanation, rather than a passive tendency to cover the prey, enlarge the birds’ aspect for defense or for threat displays.
Oddly, unlike accipiters or in fact any raptor, only the second pedal ungual is elongated, whereas the other digits are shorter-clawed. This has associated effects: The pes appears to be functionally didactyl (Xing et al., 2010; Mudroch et al., 2011), the third and fourth digits accommodating this by being parallel rather than divergent, and the fourth toe longer relative to the third than in tridactyl theropods such as Coelophysis bauri or such. It is therefore tempting to say that the functional effect of the pes (using primarily the second pedal ungual to engage the prey as an effective gripping — as in, penetrating — “talon”) differs from that of extant birds of prey; Senter (2006) even implied a defensive or agonistic function for this reason.
The hypothesis presented by Fowler et al., 2011, is thus very well presented and reasoned, and I hope to see it firmly tested with respect to smaller dromaeosaurids, especially how the pedal adaptation is affected by the large “hindwings” in Microraptor gui and Anchiornis huxleyi, which appear largely absent or unpreserved closer to the body wall and are strongly developed below the tarsus. Because large feathers approximate to the engaging area could be easily damage, it suggests either a mechanism to move these feathers out of the way, or perhaps that the foot was not actually used to engage prey in just this manner, but perhaps in a more delicate manner. It is also notable that, as preserved, juvenile Sinornithosaurus sp. (NGMC 91, or “Dave“; Ji et al., 2001), at just above 50% body size of a putative adult Sinornithosaurus millennii (Xu et al., 1999), lacks any trace of fully pennaceous feathers on its arms, while they are present perpendicular to the extended femora; adult specimens preserve no pennaceous feathers at all, only filamentous integument, as present elsewhere on the juvenile body. These may suggest that these dromaeosaurids, putative microraptorines like Microraptor gui with fully fledged feathers, may not indicate the true extent of feathered limbs, and their effective “umbra” when mantling, possible for larger-bodied dromaeosaurs. There is to date a lack of positive evidence demonstrating that large pennaceous feathers covered the arm in such a manner to enable an mantling aspect, and this is further made problematic when the tail in raptors are used to both mantle and for balance while struggling with prey (or long after the prey is dead, suggesting as I said above a possible lack of connection).
Fowler et al. (2011) propose that basal deinonychosaurs were cursors, likely pursuit hunters. I would bring attention to Hartman’s discussion of the argument that early pre-birds could run around with their arms and tail as stabilizing aerofoils, where the exaptation is towards an elevated forelimb that could tangentially lead into WAIR (Dial, 2003), and a broader, shorter tail can develop more maneuverability. Instead, the trajectory was toward an apparently hypercursorial pes in troodontids (due to proportions and the arctometatarsalian pes), while the dromaeosaur lineage shortened this feature after the split with Microraptoria. Dromaeosaurids are short-legged, larger, and likely ambush hunters. Yet it is not these animals have have developed shoulder motility, broad sterna, or proportionally longer arms to body size. They have shortened the arms, shortened the leg, and reduced shoulder mobility. Thus, it might seem that instead of developing a should-arm “flapping” mechanism on the lineage to birds, they did so completely isolated and parallel to the evolution of the modern avian flight stroke. This is important when you consider that some dromaeosaurs (esp. the unenlagiines) had particularly short forelimbs, and form the sister group to the dromaeosaurines (sensu lato, Eudromaeosauria), while at the same time retained these features at small size (e.g., Buitreraraptor gonzalezorum, an unenlagiine/unenlagiid [argh, stupid Linnaean lack of sense!]). Depending on the order of divergence (microraptorians (unenlagiines, dromaeosaurines)) or (unenlagiines (microraptorians, dromaeosaurines)), thus could upset the trajectory favored in the paper that the arms and behavior of large-bodied dromaeosaurids had anything to do with the evolution of the avian flight stroke.
I suggest, rather, that the flight stroke mechanism was present basally, and exapted into the larger-bodied forms and coupled with prey acquisition and restraint. Smaller-bodied animals are either broadly feathered and likely pursuit runners who used foot-based restraint alone, or moderate-sized pursuit runners with short arms likely unable to use anything but foot-based restraint; larger-bodied dromaeosaurs, from the “turkey sized” Velociraptor mongoliensis on up to the stocky Achillobator giganticus. It is easy to imagine these animals as grappling predators, ambush hunters, rather than pursuit runners, and that this gives great explanatory power to the disparity in limb construction, skull structure, the proportions of the limb, etc. Here‘s a prescient argument on the “stockiness” of large-bodied dromaeosaurs focusing on the short-legged, robust Achillobator giganticus by fellow “armchair” paleo nut, Matt Marynuik. He presumes, as did Fowler et al., that the stockiness is linked to the large ungual on pd2.

One can deduce, from the fantastically large ilium, robust femur and short distal limb that the muscle mass controlled a strong leg, but that the animal was not a swift one, and was probably subcursorial. Here, I show it running. I will emend this to show a more plodding [plotting?] animal, far more likely to engage in foot-to-chest combat with animals its size or larger (or smaller, at need). It is absolutely amazing to think of this in context to the amazingly short and robust limb bones of Balaur bondoc, the even stockier dromaeosaur from Romania (Csiki et al., 2009). Such an animal might indiacte even more robust, heavily clawed forelimbs than Velociraptor mongoliensis (see above). While Andrea Cau takes Balaur bondoc to task in regards to Fowler et al.’s new study here, calling Balaur “Dodoraptor,” he finds it disconforms to the general model of larger robust forms at the terminous of dromaeosaurid evolution, along with Deinonychus antirrhopus, but rather at the base of a radiation of “dromaeosaurines,” i.e., Eudromaeosauria. But I think they have yet to go far enough. It amazes me that no one has yet placed this hypothesis in comparison to those theropods with the absolutely most fabulous, amazing and bizarre feet of all, derived therizinosaurids (or “segnosaurs,” in the ancient way). Take a look:

The unguals of the pes are all mediolaterally compressed, deep, and high high dorsal curvatures. The flexor tubercles of the unguals are more moderate than in most dromaeosaurids, but not substantially more than others (see Balaur bondoc, above). The first metatarsal likely oriented medially somewhat, as indicated at least by the medial orientation of the distal articular facet to the long axis of the shaft, which when articulated is oriented medially from MTII itself. Flexibility of the digits is unknown, although strong dorsoventral compression may place the digits in the low-flexibility range of ornithomimosaurs (Senter, 2009). Despite this, the raptorial appearance of the unguals is particularly fascinating in light of both the presumed herbivorous habits of the taxa (Barsbold & Perle, 1980), but also the amazingly mediportal or graviportal posture of the body, something somewhat approached by the largest dromaeosaurids. It would be interesting to see where this plots in future analyses. If the pedal anatomy indicates a grasping structure, and the dentition is clearly herbivorous, the utility of the foot as a grasping device may divorce the function from predation, where predatory grappling may simply be a use for such a foot, but not primarily indicated by it.
In closing, I think that the proposal of Fowler et al. (2011), mantling behavior in dromaeosaurids, neatly explains the functional anatomy of the leg and arm, although I would extend this and indicate that the arms would engage the prey regularly. Thus rather than simply borrowing the “mantling hawk” motif, the arms would likely be less feathered and more engaged in prey restraint.
[n1] Bambiraptor feinbergi was coined by Burnham et al. (2000) with the intention of honoring the entire Feinberg family, whom had donated resources to enable the acquisition by the AMNH of the holotype and paratype specimens. The formation of the name feinbergi is in keeping with treatments of names honoring single individuals, but according to the International Code of Zoological Nomenclature [ICZN] (1985, 3rd edition), names honoring multiple individuals must take the form -arum (when all honored persons are female) or -orum (when at least one honored person is male), but not -i (male) or -ae (female). In keeping with this tradition, Olshevsky (2000) emended the nomenclature to feinbergorum, which has since been followed (e.g., Turner et al., 2007a and Norell & Makovicky, 2004). However, as of the release of the 4th edition of the Code (which took effect on January 1st, 2000, a date antecedent to Olshevsky, 2000 [which was released towards the middle of that year), this requirement is no longer in effect. Currently, the only spellings that must be corrected are "clear evidence of an inadverdent error, such as a lapsus calami or a copyist's or printer's error" (Art.32.5.1) or "a name published with a diacritic or other mark" (Art.32.5.2). As stressed by the Code, "ncorrect transliteration or latinization, or use of an inappropriate connecting vowel, are not to be considered inadvertent errors.” Thus, use and affirmation of “feinbergorum” as the correct spelling of the name is in error, and should be discontinued.
[n2] Dececchi & Larsson (2011) reference Mannion et al. (2009) who supported a climbing “crampon” function for the large pedal ungual, but based their analysis on a manual ungual, and Bin-Jeffrey & Rayfield (2009) [n3] refuted this. Both analyses draw particularly on comparison to extant scansorial birds, as in Fowler et al. (2009).
[n3] This is an abstract for the 2009 SVP meeting, and is thus technically “unpublished.” The citation (below) is provided solely under the basis of discussing Mannion et al. (2009) on the subject of claw function.
Abler, W. L. 1992. The serrated teeth of tyrannosaurid dinosaurs, and biting structures in other animals. Paleobiology 18(2):161-183.
Abler, W. L. 1999. The teeth of the tyrannosaurs. Scientific American 1999(9):50-51.
Abler, W. L. 2001. A kerf-and-drill model of tyrannosaur tooth serrations. pg.84-89 in Tanke, Carpenter & Skrepnick (eds.) Mesozoic Vertebrate Life: New Research Inspired by the Paleontology of Philip J. Currie. (Indiana University Press, Bloomington.)
Barsbold R. & Perle A. 1980. Segnosauria, a new infraorder of carnivorous dinosaurs. Acta Palaeontologica Polonica 25(2):187-195.
Bin-Jeffery, A. & Rayfield, E 2009. Finite element analysis of pedal claws to determine mode of life in birds, lizards and maniraptoran theropods. Journal of Vertebrate Paleontology 29(Supplement to 3):64A. [n2, see above]
Burnham, D. A., Derstler, K. L., Currie, P. J., Bakker, R. T., Zhou Z.-h. & Ostrom, J. H. 2000. Remarkable new birdlike dinosaur (Theropoda: Maniraptora) from the Upper Cretaceous of Montana. University of Kansas Paleontological Contributions, New Series 13:1–14.
Carpenter, K. 2002. Forelimb biomechanics of nonavian theropod dinosaurs in predation. Senckenbergiana Lethaea 82:59-76.
Chiappe, L. M. & Padian, K. 1998. The origin and early evolution of birds. Biological Review 73:1-42.
Currie, P. J. 1987. Bird-like characteristics of the jaws and teeth of troodontid theropods (Dinosauria, Saurischia). Journal of Vertebrate Paleontology 7(1):72-81.
Currie, P. J., Rigby, J. K., Jr. & Sloan, R. E.. 1990. Theropod teeth from the Judith River Formation of southern Alberta, Canada. pg.107-125 in Carpenter & Currie (eds.) Dinosaur Systematics: Approaches and Perspectives. (Cambridge University Press, New York City.)
Csiki, Z., Vremir, M., Brusatte, S. L. & Norell, M. A. 2010. An aberrant island-dwelling theropod dinosaur from the Late Cretaceous of Romania. Proceedings of the National Academy of Sciences of the United States of America 107 (35):15357–15361.
Dial, K. P. 2003. Wing-assisted incline running and the evolution of flight. Science 299:402–404.
Dececchi, T. A. & Larsson, H. C. E. 2011. Assessing arboreal adaptations of bird antecedents: Testing the ecological setting of the origin of the avian flight stroke. PLoS ONE 6(8):e22292.
Feduccia, A. 1993. Evidence from claw geometry indicating arboreal habits of Archaeopteryx. Science 259:790-793.
Forster, C. A., Sampson, S. D., Chiappe, L. M. & Krause, D. W. 1998. The theropod ancestry of birds: New evidence from the Late Cretaceous of Madagascar? Science 279:1915-1919.
Fowler, D. W., Freedman, E. A. & Scannella, J. B. 2009. Predatory functional morphology in raptors: Interdigital variation in talon size is related to prey restraint and immobilisation technique. PLoS ONE 4(11):e7999.
Fowler, D. W., Freedman, E. A., Scannella, J. B. & Kambic, R. E. 2011. The predatory ecology of Deinonychus and the origin of flapping in birds. PLoS ONE 6(12):e28964.
Fox, N. 1995. Development and behavior. pg.176-195 in Blaine (ed.) Understanding the Bird of Prey. (Hancock House.)
Glen, C. L. & Bennett, M. B.. 2007. Foraging modes of Mesozoic birds and non-avian theropods. Current Biology 17:R911-R912.
Hu D.-y., Hou L.-h., Zhang L.-j. & Xu X. 2009. A pre-Archaeopteryx troodontid theropod from China with long feathers on the metatarsus. Nature 461:640–643.
Ji Q., Norell, M. A., Gao, K.-q., Ji S.-a. & Ren D. 2001. The distribution of integumentary structures in a feathered dinosaur. Nature 410:1084-1087.
Longrich, N. 2006. Structure and function of hindlimb feathers in Archaeopteryx lithographica. Paleobiology 32(3):417-431.
Manning, P. L., Margetts, L., Johnson, M. R., Withers, P. J., Sellers, W. I., Falkingham, P. L., Mummery, P. M., Barrett, P. M. & Raymont, D. R. 2009. Biomechanics of dromaeosaurid dinosaur claws: Application of X-ray microtomography, nanoindentation, and finite element analysis. The Anatomical Record 292:1397–405.
Mudroch, A., Richter, U., Joger, U., Kosma, R., Idé, O. & Maga, a. 2011. Didactyl tracks of paravian theropods (Maniraptora) from the ?Middle Jurassic of Africa. PLoS ONE 6(2):e14642.
Norell, M. A. & Makovicky, P. J. 1997. Important features of the dromaeosaur skeleton: Information from a new specimen. American Museum Novitates 3215:1-28.
Norell, M. A. & Makovicky, P. J. 1999. Important features of the dromaeosaur skeleton. II. Information from newly collected specimens of Velociraptor mongoliensis. American Museum Novitates 3282:1-45.
Norell, M. A. & Makovicky, P. J. 2004. Dromaeosauridae. pg.196-209 in Weishampel, Dodson & Osmólska (eds.) The Dinosauria (2nd Edition). (University of California Press, Berkeley.)
Olshevsky, G. 2000. An annotated checklist of dinosaur species by continent. Mesozoic Meanderings 3:1-157.
Ostrom, J. H. 1969. Osteology of Deinonychus antirrhopus, an unusual theropod from the Lower Cretaceous of Montana. Bulletin of the Peabody Museum of Natural History 30:1–165.
Perle A. 1981.
?? 

?? ?? 
??
? 
?? [New segnosaurid from the Upper Cretaceous of Mongolia].
?? – 

? 
??-

?? 



?? 

?? — Joint Soviet-Mongolian Paleontological Expedition, Transactions 15:50–59.
Perle A., Marya?ska, T. & Osmólska, H. 1983. Goyocephale lattimorei gen. et sp. n., a new flat-headed pachycephalosaur (Ornithischia, dinosauria) from the Upper Cretaceous of Mongolia. Acta Palaeontologica Polonica 27(1-4):115-127.
Perle, A., Norell, M.A. & Clark, J. 1999. A new maniraptoran theropod – Achillobator giganticus (Dromaeosauridae) – from the Upper Cretaceous of Burkhant, Mongolia. Contributions of the Mongolian-American Paleontological Project 101: 1–105.
Russell, D. A. & Dong Z.-m. 1994. The affinities of a new theropod from the Alxa Desert, Inner Mongolia, People’s Republic of China. Canadian Journal of Earth Sciences — Revue canadienne de sciences de la Terre 30(10):2107-2127.
Sakamoto, M. 2010. Jaw biomechanics and the evolution of biting performance in theropod dinosaurs. Proceedings of the Royal Society B 277:3327–3333.
Senter, P. 2006. Comparison of forelimb function between Deinonychus and Bambiraptor (Theropoda: Dromaeosauridae). Journal of Vertebrate Paleontology 26(4):897-906.
Senter, P. 2009. Pedal function in deinonychosaurs (Dinosauria: Theropoda): A comparative study. Bulletin of the Gunma Museum of Natural History 13:1-14.
Sues, H.-D. 1978. A new small theropod dinosaur from the Judith River Formation (Campanian) of Alberta, Canada. Zoological Journal of the Linnaean Society 62:381-400.
Therrien, F., Henderson, D. M. & Ruff, C. B. 2005. Bite me: Biomechanical models of theropod mandibles and implications for feeding behavior. pg.179-237 in Carpenter (ed.) The Carnivorous Dinosaurs. (Indiana University Press, Bloomington.)
Turner, A. H., Hwang, S. H. & Norell, M. A. 2007a. A small derived theropod from Öösh, Early Cretaceous, Baykhangor Mongolia. American Museum Novitates 3557:1-27.
Turner, A. H., Makovicky, P. J. & Norell, M. A. 2007b. Feather quill knobs in the dinosaur Velociraptor. Science 317:1721.
Xing L.-d., Harris, J. D., Sun D.-h. & Zhao H.-q. 2009. The earliest known deinonychosaur tracks from the Jurassic-Cretaceous boundary in Hebei Province, China. Acta Palaeontologica Sinica 48(4):662-671.
Xu X., Wang X.-l. & Wu X.-c. 1999. A dromaeosaurid dinosaur with a filamentous integument from the Yixian Formation of China. Nature 401:262-266.
Xu X., Zhou Z.-h., Wang X.-l, Kuang X.-w., Zhang F.-c. & Du X.-k. 2003. Four-winged dinosaurs from China. Nature 421:335–340.
Zheng X.-t., Xu X., You H.-l., Zhao Q. & Dong Z.-m. 2010. A short-armed dromaeosaurid from the Jehol Group of China with implications for early dromaeosaurid evolution. Proceedings of the Royal Society B 277:211–217.
Jurassic Park Velociraptors are named after Velociraptor mongoliensis, and modeled after a hybrid between the genera Deinonychus and Utahraptor. At the time the novel was written, there was some talk of moving Deinonychus into the genus Velociraptor, which was the convention followed by Micheal Crichton, though this didn't play out. However, all of these were dromaeosaurids, which are eumaniraptorans. In fact, Dromaeosauridae is the group closest to but outside of Aves. So, all members of eumaniraptora have a synapomorphy list that includes a "semilunate carpal".



All three of the pictures above show this semilunate carpal in other maniraptorans. Unlike most mammalian wrists, it cannot flex perpendicular to the radius-ulna plane (ie, what we would most commonly accept as perpendicular to the groud). Instead, its motions were restricted to that plane, giving a jacknifing motion, much like a flight stroke. This was an optimal grasping motion for prey, and good for grappling large prey, and was easy to adapt into a flight stroke (particularly given the presence of primary feathers on some dromaeosaurids).
Sadly, this does mean that the raptors couldn't open doors. They would have broken their wrists trying to make the motion!
With Arms Wide Open (and Apologies to Pearl Jam)
I am very curious where this study will go next. Fowler et al. have produced a wealth of quanitified data regarding toe function and proportions (Fowler et al., 2009, 2011). These analyses attempt to place into a behavioral context the function of the dromaeosaur pes and thus feeding ecology on the whole. They took the “raptors” off the sides of the tenontosaurs and engaged them with smaller prey. Cautiously, then, one must note that this is all itself speculative. Indeed, the presence of large, flexible hands and apparent wings are themselves bizarrely-linked to the question of pedal function, as Fowler et al., 2011, themselves note:
It remains paradoxical that the manual digits of paravians seem well-suited for flexion and grasping, yet would have borne flight feathers so as to make such an action difficult or clumsy (Ostrom, 1969; Senter, 2006; Carpenter, 2002). Further, paravian manual unguals are enlarged and strong expression of flexor tubercles suggests that the claws were capable of exerting considerable force, yet the limited range of motion of the forelimb (Senter, 2006) seems strongly adapted for flapping, rather than the flexibility required for prey manipulation (or indeed, climbing (Dececchi & Larsson, 2011)).
[Fowler et al., 2011:pg.8-9; I've modified the internal reference numbers to citations, a practice of PLoS ONE's that makes internal text more legible, but reference checking more cumbersome. All cited references are included below.]
Fowler et al., 2011, primarily concern themselves with the “mid-sized” dromaeosaurids, Velociraptor mongoliensis and Deinonychus antirrhopus, but it should be noted that in the remarks above, most of the work has been to compare broadly the small nonavian theropods Microraptor zhaoianus (Dececchi & Larsson, 2011 [n2]) and Archaeopteryx lithographica (Ostrom, 1969). These analysis draw parallels based solely on the apparent articulation of the shoulder girdle, or later the apparent presence of a series of quill knobs on the particularly short ulna of Velociraptor mongoliensis (Turner et al., 2007b). While the articulation of the various elements of the shoulder are questionable (for example, check out Jason Brougham’s deconstruction of the topic at his blog, Soft Dinosaurs (and here are links to part one, two, three, four and five of this series, and an outlier discussion on dromaeosaur girdles in general, prompted by Burnham et al.’s (2000) improbably shoulder articulation for Bambiraptor feinbergi), absolute limb length implies that any mechanical leverage of the limbs and its range of motion was less than that of any extant volant-capable bird (Ostrom, 1969; Chiappe & Padian, 1998; Dial, 2003), although it was clearly greater than antecedents. It is also generally considered that Velociraptor mongoliensis and Deinonychus antirrhopus were terrestrial and non-volant in almost any fashion, yet Fowler et al., 2011, supposed they might have employed the flapping wingstroke in a form of stability flapping while engaged with prey. Thus regardless of their archaic (relative to extant birds) limb design and small pectoral and brachial muscle systems compared to, say Confuciusornis sanctus (Dececchi & Larsson, 2011), the “wings” of larger dromaeosaurids (as supposed by Fowler et al., 2011) are modeled directly after the smaller, older, and phylogenentically more basal deinonychosaurs, such as Archaeopteryx lithographica.
So what to make of the “flapping first” model versus the competing ideas? It is a compelling idea, and it takes the potential of integrating actual feeding ecology rather than circumstantial prey acquisition (such as a lizard, mammal or bird in the stomach or gut region) based on biomechanics into account. I much prefer this method of reasoning, and do not see any distinct problems with this especially in connection with WAIR (Wing-Assisted Incline Running, as proposed by Dial, 2003). It should be noted, however, that speculations on this stem directly from the largest of dromaeosaurs, not the smallest. It seems unreasonable to paint the short armed, robust and relatively shorter legged, probable ambush predators of the Djadokhta and Cloverly Formations in the same manner as the smaller “near-avians” of the Solnhofen, Yixian and Jiufotang Formations. Taking phylogenetics into account, dromaeosaurids became larger, less arboreal, as they evolved, rather than smaller and more arboreal, or more flight-capable. This implies that models based on the largest are ill-suited to help explain the smallest, or that the model can explain the unusual pedal integument of Microraptor zhaoianus, Anchiornis huxleyi or Archaeopteryx lithographica (Xu et al., 2003; Hu et al., 2009; Longrich, 2006).
These issues are actually complicated by the problems of incorporated a particularly grasping suited manus with a grasping suited pes, but relegate the actual grasping duty only to the pes, leaving the manus free. This should, on the path toward flapping or corralling behavior, lead to a stiffer manus (one of those “Just So Stories” we’ve been taught), yet the larger dromaeosaurs seem to have very flexible digits, especially the third manus digit (Senter, 2009). Moreover, it seems improbable that the arm was as winged as that of much smaller dromaeosaurs, where the wings are not broad and rounded but slender and tapering sharply (in contrast to accipiters). The tail, acting as a counter-balancing rod, seems odd in that in Microraptor gui, unlike Archaeopteryx lithographica (see Xu et al., 2003; Longrich, 2006) only the distal tip of the tail bore a “retricial” array. Absence of a distinct tail fan in raptors (any of them) implies that the distal end only structure may have had less of an effect during mantling when used to “corral” prey, than to cover it during invasive aggression of possible thieves, or threat displays. That this behavior (as Fowler et al., 2011, note) occurs when the prey is dead, the effect of maintaining purchase during the struggle may be less of an explanation, rather than a passive tendency to cover the prey, enlarge the birds’ aspect for defense or for threat displays.
Oddly, unlike accipiters or in fact any raptor, only the second pedal ungual is elongated, whereas the other digits are shorter-clawed. This has associated effects: The pes appears to be functionally didactyl (Xing et al., 2010; Mudroch et al., 2011), the third and fourth digits accommodating this by being parallel rather than divergent, and the fourth toe longer relative to the third than in tridactyl theropods such as Coelophysis bauri or such. It is therefore tempting to say that the functional effect of the pes (using primarily the second pedal ungual to engage the prey as an effective gripping — as in, penetrating — “talon”) differs from that of extant birds of prey; Senter (2006) even implied a defensive or agonistic function for this reason.
The hypothesis presented by Fowler et al., 2011, is thus very well presented and reasoned, and I hope to see it firmly tested with respect to smaller dromaeosaurids, especially how the pedal adaptation is affected by the large “hindwings” in Microraptor gui and Anchiornis huxleyi, which appear largely absent or unpreserved closer to the body wall and are strongly developed below the tarsus. Because large feathers approximate to the engaging area could be easily damage, it suggests either a mechanism to move these feathers out of the way, or perhaps that the foot was not actually used to engage prey in just this manner, but perhaps in a more delicate manner. It is also notable that, as preserved, juvenile Sinornithosaurus sp. (NGMC 91, or “Dave“; Ji et al., 2001), at just above 50% body size of a putative adult Sinornithosaurus millennii (Xu et al., 1999), lacks any trace of fully pennaceous feathers on its arms, while they are present perpendicular to the extended femora; adult specimens preserve no pennaceous feathers at all, only filamentous integument, as present elsewhere on the juvenile body. These may suggest that these dromaeosaurids, putative microraptorines like Microraptor gui with fully fledged feathers, may not indicate the true extent of feathered limbs, and their effective “umbra” when mantling, possible for larger-bodied dromaeosaurs. There is to date a lack of positive evidence demonstrating that large pennaceous feathers covered the arm in such a manner to enable an mantling aspect, and this is further made problematic when the tail in raptors are used to both mantle and for balance while struggling with prey (or long after the prey is dead, suggesting as I said above a possible lack of connection).
Fowler et al. (2011) propose that basal deinonychosaurs were cursors, likely pursuit hunters. I would bring attention to Hartman’s discussion of the argument that early pre-birds could run around with their arms and tail as stabilizing aerofoils, where the exaptation is towards an elevated forelimb that could tangentially lead into WAIR (Dial, 2003), and a broader, shorter tail can develop more maneuverability. Instead, the trajectory was toward an apparently hypercursorial pes in troodontids (due to proportions and the arctometatarsalian pes), while the dromaeosaur lineage shortened this feature after the split with Microraptoria. Dromaeosaurids are short-legged, larger, and likely ambush hunters. Yet it is not these animals have have developed shoulder motility, broad sterna, or proportionally longer arms to body size. They have shortened the arms, shortened the leg, and reduced shoulder mobility. Thus, it might seem that instead of developing a should-arm “flapping” mechanism on the lineage to birds, they did so completely isolated and parallel to the evolution of the modern avian flight stroke. This is important when you consider that some dromaeosaurs (esp. the unenlagiines) had particularly short forelimbs, and form the sister group to the dromaeosaurines (sensu lato, Eudromaeosauria), while at the same time retained these features at small size (e.g., Buitreraraptor gonzalezorum, an unenlagiine/unenlagiid [argh, stupid Linnaean lack of sense!]). Depending on the order of divergence (microraptorians (unenlagiines, dromaeosaurines)) or (unenlagiines (microraptorians, dromaeosaurines)), thus could upset the trajectory favored in the paper that the arms and behavior of large-bodied dromaeosaurids had anything to do with the evolution of the avian flight stroke.
I suggest, rather, that the flight stroke mechanism was present basally, and exapted into the larger-bodied forms and coupled with prey acquisition and restraint. Smaller-bodied animals are either broadly feathered and likely pursuit runners who used foot-based restraint alone, or moderate-sized pursuit runners with short arms likely unable to use anything but foot-based restraint; larger-bodied dromaeosaurs, from the “turkey sized” Velociraptor mongoliensis on up to the stocky Achillobator giganticus. It is easy to imagine these animals as grappling predators, ambush hunters, rather than pursuit runners, and that this gives great explanatory power to the disparity in limb construction, skull structure, the proportions of the limb, etc. Here‘s a prescient argument on the “stockiness” of large-bodied dromaeosaurs focusing on the short-legged, robust Achillobator giganticus by fellow “armchair” paleo nut, Matt Marynuik. He presumes, as did Fowler et al., that the stockiness is linked to the large ungual on pd2.

One can deduce, from the fantastically large ilium, robust femur and short distal limb that the muscle mass controlled a strong leg, but that the animal was not a swift one, and was probably subcursorial. Here, I show it running. I will emend this to show a more plodding [plotting?] animal, far more likely to engage in foot-to-chest combat with animals its size or larger (or smaller, at need). It is absolutely amazing to think of this in context to the amazingly short and robust limb bones of Balaur bondoc, the even stockier dromaeosaur from Romania (Csiki et al., 2009). Such an animal might indiacte even more robust, heavily clawed forelimbs than Velociraptor mongoliensis (see above). While Andrea Cau takes Balaur bondoc to task in regards to Fowler et al.’s new study here, calling Balaur “Dodoraptor,” he finds it disconforms to the general model of larger robust forms at the terminous of dromaeosaurid evolution, along with Deinonychus antirrhopus, but rather at the base of a radiation of “dromaeosaurines,” i.e., Eudromaeosauria. But I think they have yet to go far enough. It amazes me that no one has yet placed this hypothesis in comparison to those theropods with the absolutely most fabulous, amazing and bizarre feet of all, derived therizinosaurids (or “segnosaurs,” in the ancient way). Take a look:

The unguals of the pes are all mediolaterally compressed, deep, and high high dorsal curvatures. The flexor tubercles of the unguals are more moderate than in most dromaeosaurids, but not substantially more than others (see Balaur bondoc, above). The first metatarsal likely oriented medially somewhat, as indicated at least by the medial orientation of the distal articular facet to the long axis of the shaft, which when articulated is oriented medially from MTII itself. Flexibility of the digits is unknown, although strong dorsoventral compression may place the digits in the low-flexibility range of ornithomimosaurs (Senter, 2009). Despite this, the raptorial appearance of the unguals is particularly fascinating in light of both the presumed herbivorous habits of the taxa (Barsbold & Perle, 1980), but also the amazingly mediportal or graviportal posture of the body, something somewhat approached by the largest dromaeosaurids. It would be interesting to see where this plots in future analyses. If the pedal anatomy indicates a grasping structure, and the dentition is clearly herbivorous, the utility of the foot as a grasping device may divorce the function from predation, where predatory grappling may simply be a use for such a foot, but not primarily indicated by it.
In closing, I think that the proposal of Fowler et al. (2011), mantling behavior in dromaeosaurids, neatly explains the functional anatomy of the leg and arm, although I would extend this and indicate that the arms would engage the prey regularly. Thus rather than simply borrowing the “mantling hawk” motif, the arms would likely be less feathered and more engaged in prey restraint.
[n1] Bambiraptor feinbergi was coined by Burnham et al. (2000) with the intention of honoring the entire Feinberg family, whom had donated resources to enable the acquisition by the AMNH of the holotype and paratype specimens. The formation of the name feinbergi is in keeping with treatments of names honoring single individuals, but according to the International Code of Zoological Nomenclature [ICZN] (1985, 3rd edition), names honoring multiple individuals must take the form -arum (when all honored persons are female) or -orum (when at least one honored person is male), but not -i (male) or -ae (female). In keeping with this tradition, Olshevsky (2000) emended the nomenclature to feinbergorum, which has since been followed (e.g., Turner et al., 2007a and Norell & Makovicky, 2004). However, as of the release of the 4th edition of the Code (which took effect on January 1st, 2000, a date antecedent to Olshevsky, 2000 [which was released towards the middle of that year), this requirement is no longer in effect. Currently, the only spellings that must be corrected are "clear evidence of an inadverdent error, such as a lapsus calami or a copyist's or printer's error" (Art.32.5.1) or "a name published with a diacritic or other mark" (Art.32.5.2). As stressed by the Code, "ncorrect transliteration or latinization, or use of an inappropriate connecting vowel, are not to be considered inadvertent errors.” Thus, use and affirmation of “feinbergorum” as the correct spelling of the name is in error, and should be discontinued.
[n2] Dececchi & Larsson (2011) reference Mannion et al. (2009) who supported a climbing “crampon” function for the large pedal ungual, but based their analysis on a manual ungual, and Bin-Jeffrey & Rayfield (2009) [n3] refuted this. Both analyses draw particularly on comparison to extant scansorial birds, as in Fowler et al. (2009).
[n3] This is an abstract for the 2009 SVP meeting, and is thus technically “unpublished.” The citation (below) is provided solely under the basis of discussing Mannion et al. (2009) on the subject of claw function.
Abler, W. L. 1992. The serrated teeth of tyrannosaurid dinosaurs, and biting structures in other animals. Paleobiology 18(2):161-183.
Abler, W. L. 1999. The teeth of the tyrannosaurs. Scientific American 1999(9):50-51.
Abler, W. L. 2001. A kerf-and-drill model of tyrannosaur tooth serrations. pg.84-89 in Tanke, Carpenter & Skrepnick (eds.) Mesozoic Vertebrate Life: New Research Inspired by the Paleontology of Philip J. Currie. (Indiana University Press, Bloomington.)
Barsbold R. & Perle A. 1980. Segnosauria, a new infraorder of carnivorous dinosaurs. Acta Palaeontologica Polonica 25(2):187-195.
Bin-Jeffery, A. & Rayfield, E 2009. Finite element analysis of pedal claws to determine mode of life in birds, lizards and maniraptoran theropods. Journal of Vertebrate Paleontology 29(Supplement to 3):64A. [n2, see above]
Burnham, D. A., Derstler, K. L., Currie, P. J., Bakker, R. T., Zhou Z.-h. & Ostrom, J. H. 2000. Remarkable new birdlike dinosaur (Theropoda: Maniraptora) from the Upper Cretaceous of Montana. University of Kansas Paleontological Contributions, New Series 13:1–14.
Carpenter, K. 2002. Forelimb biomechanics of nonavian theropod dinosaurs in predation. Senckenbergiana Lethaea 82:59-76.
Chiappe, L. M. & Padian, K. 1998. The origin and early evolution of birds. Biological Review 73:1-42.
Currie, P. J. 1987. Bird-like characteristics of the jaws and teeth of troodontid theropods (Dinosauria, Saurischia). Journal of Vertebrate Paleontology 7(1):72-81.
Currie, P. J., Rigby, J. K., Jr. & Sloan, R. E.. 1990. Theropod teeth from the Judith River Formation of southern Alberta, Canada. pg.107-125 in Carpenter & Currie (eds.) Dinosaur Systematics: Approaches and Perspectives. (Cambridge University Press, New York City.)
Csiki, Z., Vremir, M., Brusatte, S. L. & Norell, M. A. 2010. An aberrant island-dwelling theropod dinosaur from the Late Cretaceous of Romania. Proceedings of the National Academy of Sciences of the United States of America 107 (35):15357–15361.
Dial, K. P. 2003. Wing-assisted incline running and the evolution of flight. Science 299:402–404.
Dececchi, T. A. & Larsson, H. C. E. 2011. Assessing arboreal adaptations of bird antecedents: Testing the ecological setting of the origin of the avian flight stroke. PLoS ONE 6(8):e22292.
Feduccia, A. 1993. Evidence from claw geometry indicating arboreal habits of Archaeopteryx. Science 259:790-793.
Forster, C. A., Sampson, S. D., Chiappe, L. M. & Krause, D. W. 1998. The theropod ancestry of birds: New evidence from the Late Cretaceous of Madagascar? Science 279:1915-1919.
Fowler, D. W., Freedman, E. A. & Scannella, J. B. 2009. Predatory functional morphology in raptors: Interdigital variation in talon size is related to prey restraint and immobilisation technique. PLoS ONE 4(11):e7999.
Fowler, D. W., Freedman, E. A., Scannella, J. B. & Kambic, R. E. 2011. The predatory ecology of Deinonychus and the origin of flapping in birds. PLoS ONE 6(12):e28964.
Fox, N. 1995. Development and behavior. pg.176-195 in Blaine (ed.) Understanding the Bird of Prey. (Hancock House.)
Glen, C. L. & Bennett, M. B.. 2007. Foraging modes of Mesozoic birds and non-avian theropods. Current Biology 17:R911-R912.
Hu D.-y., Hou L.-h., Zhang L.-j. & Xu X. 2009. A pre-Archaeopteryx troodontid theropod from China with long feathers on the metatarsus. Nature 461:640–643.
Ji Q., Norell, M. A., Gao, K.-q., Ji S.-a. & Ren D. 2001. The distribution of integumentary structures in a feathered dinosaur. Nature 410:1084-1087.
Longrich, N. 2006. Structure and function of hindlimb feathers in Archaeopteryx lithographica. Paleobiology 32(3):417-431.
Manning, P. L., Margetts, L., Johnson, M. R., Withers, P. J., Sellers, W. I., Falkingham, P. L., Mummery, P. M., Barrett, P. M. & Raymont, D. R. 2009. Biomechanics of dromaeosaurid dinosaur claws: Application of X-ray microtomography, nanoindentation, and finite element analysis. The Anatomical Record 292:1397–405.
Mudroch, A., Richter, U., Joger, U., Kosma, R., Idé, O. & Maga, a. 2011. Didactyl tracks of paravian theropods (Maniraptora) from the ?Middle Jurassic of Africa. PLoS ONE 6(2):e14642.
Norell, M. A. & Makovicky, P. J. 1997. Important features of the dromaeosaur skeleton: Information from a new specimen. American Museum Novitates 3215:1-28.
Norell, M. A. & Makovicky, P. J. 1999. Important features of the dromaeosaur skeleton. II. Information from newly collected specimens of Velociraptor mongoliensis. American Museum Novitates 3282:1-45.
Norell, M. A. & Makovicky, P. J. 2004. Dromaeosauridae. pg.196-209 in Weishampel, Dodson & Osmólska (eds.) The Dinosauria (2nd Edition). (University of California Press, Berkeley.)
Olshevsky, G. 2000. An annotated checklist of dinosaur species by continent. Mesozoic Meanderings 3:1-157.
Ostrom, J. H. 1969. Osteology of Deinonychus antirrhopus, an unusual theropod from the Lower Cretaceous of Montana. Bulletin of the Peabody Museum of Natural History 30:1–165.
Perle A. 1981.


























Perle A., Marya?ska, T. & Osmólska, H. 1983. Goyocephale lattimorei gen. et sp. n., a new flat-headed pachycephalosaur (Ornithischia, dinosauria) from the Upper Cretaceous of Mongolia. Acta Palaeontologica Polonica 27(1-4):115-127.
Perle, A., Norell, M.A. & Clark, J. 1999. A new maniraptoran theropod – Achillobator giganticus (Dromaeosauridae) – from the Upper Cretaceous of Burkhant, Mongolia. Contributions of the Mongolian-American Paleontological Project 101: 1–105.
Russell, D. A. & Dong Z.-m. 1994. The affinities of a new theropod from the Alxa Desert, Inner Mongolia, People’s Republic of China. Canadian Journal of Earth Sciences — Revue canadienne de sciences de la Terre 30(10):2107-2127.
Sakamoto, M. 2010. Jaw biomechanics and the evolution of biting performance in theropod dinosaurs. Proceedings of the Royal Society B 277:3327–3333.
Senter, P. 2006. Comparison of forelimb function between Deinonychus and Bambiraptor (Theropoda: Dromaeosauridae). Journal of Vertebrate Paleontology 26(4):897-906.
Senter, P. 2009. Pedal function in deinonychosaurs (Dinosauria: Theropoda): A comparative study. Bulletin of the Gunma Museum of Natural History 13:1-14.
Sues, H.-D. 1978. A new small theropod dinosaur from the Judith River Formation (Campanian) of Alberta, Canada. Zoological Journal of the Linnaean Society 62:381-400.
Therrien, F., Henderson, D. M. & Ruff, C. B. 2005. Bite me: Biomechanical models of theropod mandibles and implications for feeding behavior. pg.179-237 in Carpenter (ed.) The Carnivorous Dinosaurs. (Indiana University Press, Bloomington.)
Turner, A. H., Hwang, S. H. & Norell, M. A. 2007a. A small derived theropod from Öösh, Early Cretaceous, Baykhangor Mongolia. American Museum Novitates 3557:1-27.
Turner, A. H., Makovicky, P. J. & Norell, M. A. 2007b. Feather quill knobs in the dinosaur Velociraptor. Science 317:1721.
Xing L.-d., Harris, J. D., Sun D.-h. & Zhao H.-q. 2009. The earliest known deinonychosaur tracks from the Jurassic-Cretaceous boundary in Hebei Province, China. Acta Palaeontologica Sinica 48(4):662-671.
Xu X., Wang X.-l. & Wu X.-c. 1999. A dromaeosaurid dinosaur with a filamentous integument from the Yixian Formation of China. Nature 401:262-266.
Xu X., Zhou Z.-h., Wang X.-l, Kuang X.-w., Zhang F.-c. & Du X.-k. 2003. Four-winged dinosaurs from China. Nature 421:335–340.
Zheng X.-t., Xu X., You H.-l., Zhao Q. & Dong Z.-m. 2010. A short-armed dromaeosaurid from the Jehol Group of China with implications for early dromaeosaurid evolution. Proceedings of the Royal Society B 277:211–217.